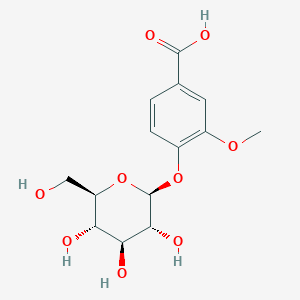
Vanillic acid glucoside
Overview
Description
Vanillic acid glucoside is a phenolic glucoside compound that can be isolated from natural sources such as fennel seeds . It is characterized by its white to off-white solid appearance and has a molecular formula of C13H16O8 . This compound is known for its potential biological activities and is used in various scientific research applications.
Mechanism of Action
Target of Action
It’s known that vanillic acid, a related compound, has been shown to interact with various cellular targets, including pancreatic β-cells , and it has been associated with the regulation of c-Jun N-Terminal Kinase in the brain .
Mode of Action
Studies on vanillic acid have shown that it can potentiate insulin secretion and prevent pancreatic β-cells cytotoxicity under h2o2-induced oxidative stress . It also appears to regulate c-Jun N-Terminal Kinase in the brain, which may have implications for neurotoxicity .
Biochemical Pathways
Vanillic acid has been shown to affect several pathways, including the adenosine monophosphate-activated protein kinase (ampk), nuclear factor kappa b (nf- κb), the janus kinase (jak)/signal transducer and activator of transcription (stat), nod128;like receptor family protein (nlrp), toll-like receptors (tlrs), mitogen-activated signaling proteins (mapk) and mammalian target of rapamycin (mtor) signaling pathways .
Pharmacokinetics
A study on vanillic acid showed that it has a half-life of 05 to 96 hours, with peak serum concentrations occurring between 2 and 30 hours post-consumption .
Result of Action
Vanillic acid has been shown to protect pancreatic β-cells from h2o2-induced oxidative stress and potentiate insulin secretion . It also appears to have neuroprotective effects .
Action Environment
It’s known that the conversion of ferulic acid to vanillin, a related compound, can be significantly reduced when the substrate concentration is higher than 08 g/L .
Preparation Methods
Synthetic Routes and Reaction Conditions
The synthesis of Vanillic acid glucoside typically involves the glycosylation of 3-methoxybenzoic acid with a suitable glucopyranosyl donor. The reaction is often catalyzed by an acid or enzyme to facilitate the formation of the glycosidic bond. The reaction conditions may include:
Temperature: Typically carried out at room temperature or slightly elevated temperatures.
Solvent: Common solvents include methanol, ethanol, or water.
Catalyst: Acid catalysts such as hydrochloric acid or sulfuric acid, or enzymatic catalysts like glucosidases.
Industrial Production Methods
Industrial production of this compound may involve large-scale extraction from natural sources or chemical synthesis using optimized reaction conditions to ensure high yield and purity. The process may include:
Extraction: Isolation from plant materials using solvents like ethanol or methanol.
Purification: Techniques such as crystallization, chromatography, or recrystallization to obtain the pure compound.
Chemical Reactions Analysis
Types of Reactions
Vanillic acid glucoside undergoes various chemical reactions, including:
Oxidation: The phenolic group can be oxidized to form quinones.
Reduction: The carboxylic acid group can be reduced to form alcohols.
Substitution: The methoxy group can undergo nucleophilic substitution reactions.
Common Reagents and Conditions
Oxidation: Reagents such as potassium permanganate or hydrogen peroxide under acidic or basic conditions.
Reduction: Reagents like lithium aluminum hydride or sodium borohydride.
Substitution: Nucleophiles such as hydroxide ions or amines.
Major Products Formed
Oxidation: Formation of quinones.
Reduction: Formation of alcohol derivatives.
Substitution: Formation of substituted benzoic acid derivatives.
Scientific Research Applications
Vanillic acid glucoside has a wide range of scientific research applications, including:
Chemistry: Used as a model compound for studying glycosylation reactions and phenolic compound behavior.
Biology: Investigated for its potential antioxidant and antimicrobial properties.
Medicine: Explored for its potential therapeutic effects, including anti-inflammatory and anticancer activities.
Industry: Utilized in the development of natural product-based pharmaceuticals and nutraceuticals.
Comparison with Similar Compounds
Similar Compounds
- 4-(beta-D-Glucopyranosyloxy)benzoic acid
- 3-(beta-D-Glucopyranosyloxy)benzoic acid
- 4-(beta-D-Glucopyranosyloxy)-2-methoxybenzoic acid
Uniqueness
Vanillic acid glucoside is unique due to its specific substitution pattern on the benzoic acid ring, which influences its chemical reactivity and biological activity. The presence of the methoxy group at the 3-position and the glucopyranosyloxy group at the 4-position provides distinct properties compared to other similar compounds.
Properties
IUPAC Name |
3-methoxy-4-[(2S,3R,4S,5S,6R)-3,4,5-trihydroxy-6-(hydroxymethyl)oxan-2-yl]oxybenzoic acid | |
---|---|---|
Source | PubChem | |
URL | https://pubchem.ncbi.nlm.nih.gov | |
Description | Data deposited in or computed by PubChem | |
InChI |
InChI=1S/C14H18O9/c1-21-8-4-6(13(19)20)2-3-7(8)22-14-12(18)11(17)10(16)9(5-15)23-14/h2-4,9-12,14-18H,5H2,1H3,(H,19,20)/t9-,10-,11+,12-,14-/m1/s1 | |
Source | PubChem | |
URL | https://pubchem.ncbi.nlm.nih.gov | |
Description | Data deposited in or computed by PubChem | |
InChI Key |
JYFOSWJYZIVJPO-YGEZULPYSA-N | |
Source | PubChem | |
URL | https://pubchem.ncbi.nlm.nih.gov | |
Description | Data deposited in or computed by PubChem | |
Canonical SMILES |
COC1=C(C=CC(=C1)C(=O)O)OC2C(C(C(C(O2)CO)O)O)O | |
Source | PubChem | |
URL | https://pubchem.ncbi.nlm.nih.gov | |
Description | Data deposited in or computed by PubChem | |
Isomeric SMILES |
COC1=C(C=CC(=C1)C(=O)O)O[C@H]2[C@@H]([C@H]([C@@H]([C@H](O2)CO)O)O)O | |
Source | PubChem | |
URL | https://pubchem.ncbi.nlm.nih.gov | |
Description | Data deposited in or computed by PubChem | |
Molecular Formula |
C14H18O9 | |
Source | PubChem | |
URL | https://pubchem.ncbi.nlm.nih.gov | |
Description | Data deposited in or computed by PubChem | |
DSSTOX Substance ID |
DTXSID80556358 | |
Record name | 4-(beta-D-Glucopyranosyloxy)-3-methoxybenzoic acid | |
Source | EPA DSSTox | |
URL | https://comptox.epa.gov/dashboard/DTXSID80556358 | |
Description | DSSTox provides a high quality public chemistry resource for supporting improved predictive toxicology. | |
Molecular Weight |
330.29 g/mol | |
Source | PubChem | |
URL | https://pubchem.ncbi.nlm.nih.gov | |
Description | Data deposited in or computed by PubChem | |
CAS No. |
32142-31-7 | |
Record name | 4-(beta-D-Glucopyranosyloxy)-3-methoxybenzoic acid | |
Source | EPA DSSTox | |
URL | https://comptox.epa.gov/dashboard/DTXSID80556358 | |
Description | DSSTox provides a high quality public chemistry resource for supporting improved predictive toxicology. | |
Retrosynthesis Analysis
AI-Powered Synthesis Planning: Our tool employs the Template_relevance Pistachio, Template_relevance Bkms_metabolic, Template_relevance Pistachio_ringbreaker, Template_relevance Reaxys, Template_relevance Reaxys_biocatalysis model, leveraging a vast database of chemical reactions to predict feasible synthetic routes.
One-Step Synthesis Focus: Specifically designed for one-step synthesis, it provides concise and direct routes for your target compounds, streamlining the synthesis process.
Accurate Predictions: Utilizing the extensive PISTACHIO, BKMS_METABOLIC, PISTACHIO_RINGBREAKER, REAXYS, REAXYS_BIOCATALYSIS database, our tool offers high-accuracy predictions, reflecting the latest in chemical research and data.
Strategy Settings
Precursor scoring | Relevance Heuristic |
---|---|
Min. plausibility | 0.01 |
Model | Template_relevance |
Template Set | Pistachio/Bkms_metabolic/Pistachio_ringbreaker/Reaxys/Reaxys_biocatalysis |
Top-N result to add to graph | 6 |
Feasible Synthetic Routes
Q1: What are the main sources of Vanillic Acid Glucoside and what are its potential health benefits?
A1: this compound (4-(beta-D-Glucopyranosyloxy)-3-methoxybenzoic acid) has been identified in various plant sources. For instance, it's been found in kiwi fruit (Actinidia deliciosa) [], specifically in the shell extracts where it contributes to the significant antioxidant activity observed []. Additionally, it's been isolated from the parasitic plant Orobanche foetida where it exhibits hepatoprotective potential by countering liver damage induced by CCl4 in rats []. This protective effect is linked to its antioxidant and anti-inflammatory properties, potentially through strong binding interactions with inflammatory proteins [].
Q2: How does this compound contribute to the sensory perception of certain foods?
A2: Research indicates that this compound plays a role in the somatosensory properties of canned prunes (Prunus domestica) []. Specifically, it contributes to the perception of "slippery" and "thickness" sensations []. This suggests that this compound, alongside other phenolic compounds, could be manipulated in food products to achieve desired textural experiences.
Q3: Can you elaborate on the antioxidant properties of this compound and its potential mechanism of action?
A3: this compound, alongside other phenolic compounds, contributes to the high antioxidant activity found in kiwi fruit shell extracts []. While the exact mechanisms were not fully elucidated in the provided research, its antioxidant capacity is likely related to its ability to scavenge free radicals and chelate metal ions, similar to other phenolic compounds []. Further research on Orobanche foetida supports this, demonstrating that this compound exhibits substantial antioxidant potential in DPPH and ABTS assays []. This activity is suggested to be linked to its binding affinity for inflammatory proteins, potentially hindering their activity and downstream inflammatory pathways [].
Q4: Are there any studies exploring the structure-activity relationship of this compound?
A4: While the provided research doesn't delve into specific structure-activity relationship studies for this compound, it highlights the compound's presence alongside other phenolic compounds with known antioxidant properties, such as caffeoylquinic acids and proanthocyanidins, in plums (Prunus domestica and Prunus salicina) and other plant sources [, ]. Investigating how structural variations within this class of compounds, including the glucoside moiety of this compound, influence their antioxidant activity and other biological effects could be a promising avenue for future research.
Q5: What analytical techniques are commonly employed for the identification and quantification of this compound in plant materials and food products?
A5: Researchers utilize a range of analytical techniques to identify and quantify this compound. High-performance liquid chromatography coupled with mass spectrometry (HPLC-MS/MS) has been instrumental in characterizing phenolic compounds, including this compound, in various matrices such as plums (Prunus salicina, Prunus domestica) and Orobanche foetida [, ]. This technique allows for the separation and identification of individual compounds based on their mass-to-charge ratio and fragmentation patterns. Further quantitative analysis can be achieved by comparing peak areas against known standards.
Disclaimer and Information on In-Vitro Research Products
Please be aware that all articles and product information presented on BenchChem are intended solely for informational purposes. The products available for purchase on BenchChem are specifically designed for in-vitro studies, which are conducted outside of living organisms. In-vitro studies, derived from the Latin term "in glass," involve experiments performed in controlled laboratory settings using cells or tissues. It is important to note that these products are not categorized as medicines or drugs, and they have not received approval from the FDA for the prevention, treatment, or cure of any medical condition, ailment, or disease. We must emphasize that any form of bodily introduction of these products into humans or animals is strictly prohibited by law. It is essential to adhere to these guidelines to ensure compliance with legal and ethical standards in research and experimentation.