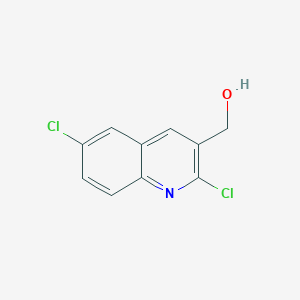
2,6-Dichloroquinoline-3-methanol
Overview
Description
2,6-Dichloroquinoline-3-methanol is a chemical compound with the empirical formula C10H7Cl2NO . It is a solid substance and is used for proteomics research .
Molecular Structure Analysis
2,6-Dichloroquinoline-3-methanol contains a total of 22 bonds; 15 non-H bonds, 11 multiple bonds, 1 rotatable bond, 11 aromatic bonds, 2 six-membered rings, 1 ten-membered ring, 1 hydroxyl group, 1 primary alcohol, and 1 Pyridine .Physical And Chemical Properties Analysis
2,6-Dichloroquinoline-3-methanol is a solid substance . It has a molecular weight of 228.07 . The SMILES string for this compound is OCc1cc2cc(Cl)ccc2nc1Cl .Scientific Research Applications
Lipid Dynamics in Biological Membranes:
- Methanol, a common solubilizing agent in biological and synthetic membranes, significantly impacts lipid dynamics. Methanol accelerates the mixing, transfer, and flip-flop kinetics of 1,2-dimyristoyl-sn-glycero-3-phosphocholine in large unilamellar vesicles, influencing the structure-function relationship of the bilayer, which is crucial for cell survival and protein reconstitution (Nguyen et al., 2019).
Antimalarial Compounds Synthesis:
- Research on antimalarial compounds has led to the synthesis of various quinoline amino alcohols, demonstrating efficacy against P. berghei in mice. Such compounds highlight the potential of quinoline derivatives in medical applications (Lutz & Sanders, 1976).
Synthesis of N-Heterocycles:
- Methanol has been used as a C1 source in the electrocatalytic synthesis of 2,3-dihydroquinazolin-4(1H)-one, a process that can proceed without external oxidants or bases. This highlights methanol's role in facilitating the synthesis of important N-heterocycles (Liu, Xu, & Wei, 2021).
Intermolecular Interactions in Quinolines:
- Research on 2-chloro-3-quinolinyl methanol revealed the nature of Cl···Cl intermolecular interactions and how they correlate with the geometry and polar flattening effects, significant in understanding molecular interactions and design (Hathwar & Guru Row, 2010).
Selective Fluorescent Chemosensors:
- 8-Hydroxyquinoline-based chemosensors in aqueous methanol solutions have shown selective fluorescence enhancement for Cd(2+), emphasizing the role of quinoline derivatives in developing sensitive detection tools for specific metal ions (Tang et al., 2008).
Mechanistic Studies in Organic Reactions:
- The role of methanol as a solvent has been studied in various copper-catalyzed oxidative coupling reactions, highlighting how solvent choice can influence reaction pathways and the stabilization of reaction intermediates (Boess et al., 2011).
Safety and Hazards
properties
IUPAC Name |
(2,6-dichloroquinolin-3-yl)methanol | |
---|---|---|
Source | PubChem | |
URL | https://pubchem.ncbi.nlm.nih.gov | |
Description | Data deposited in or computed by PubChem | |
InChI |
InChI=1S/C10H7Cl2NO/c11-8-1-2-9-6(4-8)3-7(5-14)10(12)13-9/h1-4,14H,5H2 | |
Source | PubChem | |
URL | https://pubchem.ncbi.nlm.nih.gov | |
Description | Data deposited in or computed by PubChem | |
InChI Key |
RGVUMJRZROPQIV-UHFFFAOYSA-N | |
Source | PubChem | |
URL | https://pubchem.ncbi.nlm.nih.gov | |
Description | Data deposited in or computed by PubChem | |
Canonical SMILES |
C1=CC2=NC(=C(C=C2C=C1Cl)CO)Cl | |
Source | PubChem | |
URL | https://pubchem.ncbi.nlm.nih.gov | |
Description | Data deposited in or computed by PubChem | |
Molecular Formula |
C10H7Cl2NO | |
Source | PubChem | |
URL | https://pubchem.ncbi.nlm.nih.gov | |
Description | Data deposited in or computed by PubChem | |
DSSTOX Substance ID |
DTXSID30443441 | |
Record name | 2,6-Dichloroquinoline-3-methanol | |
Source | EPA DSSTox | |
URL | https://comptox.epa.gov/dashboard/DTXSID30443441 | |
Description | DSSTox provides a high quality public chemistry resource for supporting improved predictive toxicology. | |
Molecular Weight |
228.07 g/mol | |
Source | PubChem | |
URL | https://pubchem.ncbi.nlm.nih.gov | |
Description | Data deposited in or computed by PubChem | |
Product Name |
2,6-Dichloroquinoline-3-methanol | |
CAS RN |
1017429-35-4 | |
Record name | 2,6-Dichloroquinoline-3-methanol | |
Source | EPA DSSTox | |
URL | https://comptox.epa.gov/dashboard/DTXSID30443441 | |
Description | DSSTox provides a high quality public chemistry resource for supporting improved predictive toxicology. | |
Record name | 1017429-35-4 | |
Source | European Chemicals Agency (ECHA) | |
URL | https://echa.europa.eu/information-on-chemicals | |
Description | The European Chemicals Agency (ECHA) is an agency of the European Union which is the driving force among regulatory authorities in implementing the EU's groundbreaking chemicals legislation for the benefit of human health and the environment as well as for innovation and competitiveness. | |
Explanation | Use of the information, documents and data from the ECHA website is subject to the terms and conditions of this Legal Notice, and subject to other binding limitations provided for under applicable law, the information, documents and data made available on the ECHA website may be reproduced, distributed and/or used, totally or in part, for non-commercial purposes provided that ECHA is acknowledged as the source: "Source: European Chemicals Agency, http://echa.europa.eu/". Such acknowledgement must be included in each copy of the material. ECHA permits and encourages organisations and individuals to create links to the ECHA website under the following cumulative conditions: Links can only be made to webpages that provide a link to the Legal Notice page. | |
Retrosynthesis Analysis
AI-Powered Synthesis Planning: Our tool employs the Template_relevance Pistachio, Template_relevance Bkms_metabolic, Template_relevance Pistachio_ringbreaker, Template_relevance Reaxys, Template_relevance Reaxys_biocatalysis model, leveraging a vast database of chemical reactions to predict feasible synthetic routes.
One-Step Synthesis Focus: Specifically designed for one-step synthesis, it provides concise and direct routes for your target compounds, streamlining the synthesis process.
Accurate Predictions: Utilizing the extensive PISTACHIO, BKMS_METABOLIC, PISTACHIO_RINGBREAKER, REAXYS, REAXYS_BIOCATALYSIS database, our tool offers high-accuracy predictions, reflecting the latest in chemical research and data.
Strategy Settings
Precursor scoring | Relevance Heuristic |
---|---|
Min. plausibility | 0.01 |
Model | Template_relevance |
Template Set | Pistachio/Bkms_metabolic/Pistachio_ringbreaker/Reaxys/Reaxys_biocatalysis |
Top-N result to add to graph | 6 |
Feasible Synthetic Routes
Disclaimer and Information on In-Vitro Research Products
Please be aware that all articles and product information presented on BenchChem are intended solely for informational purposes. The products available for purchase on BenchChem are specifically designed for in-vitro studies, which are conducted outside of living organisms. In-vitro studies, derived from the Latin term "in glass," involve experiments performed in controlled laboratory settings using cells or tissues. It is important to note that these products are not categorized as medicines or drugs, and they have not received approval from the FDA for the prevention, treatment, or cure of any medical condition, ailment, or disease. We must emphasize that any form of bodily introduction of these products into humans or animals is strictly prohibited by law. It is essential to adhere to these guidelines to ensure compliance with legal and ethical standards in research and experimentation.