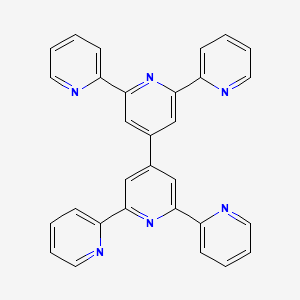
Bisterpy
Overview
Description
Bisterpy is a chemical compound that has been gaining attention in the scientific community due to its potential applications in research. It is a heterocyclic compound with a molecular formula of C10H8N2O2 and a molecular weight of 196.18 g/mol. This compound is commonly used in the synthesis of organic compounds and has been found to have various biochemical and physiological effects. In
Scientific Research Applications
Metal-Mediated Coordination Polyelectrolyte Hybrids
The self-assembly of metal-mediated coordination polyelectrolyte multilayers employing a bisterpyridine ligand (Bisterpy) has been explored at air-water interfaces. These assemblies are facilitated by coordination reactions between the bidentate ligand this compound and inorganic salts, supported by anionic poly(styrenesulfonic acid-o-maleic) (PSS) acid layers to avoid dissolution. Such hybrids demonstrate potential in the development of redox-based molecular switches and display devices, attributed to their strong stability and electrochromic response under applied potentials, showcasing this compound's role in facilitating advanced material functionalities (Zhang et al., 2009).
Self-Healing Polymer Coatings
This compound has been integrated into self-healing polymer coatings through iron bisterpyridine complexes embedded in a polymer network based on methacrylates. These materials exhibit self-healing properties, with the influence of comonomers on thermal properties being a focal point of study. This application underscores the potential of this compound in creating advanced materials that can repair themselves, contributing to longer-lasting and more sustainable material solutions (Bode et al., 2013).
Nanohybrid Construction
The complexation of multiwalled carbon nanotubes (MWNTs) with novel hexameric metallomacrocycles using a functionalized bis(terpyridine) building block represents another significant application of this compound. This method produces nanohybrid adducts characterized by transmission electron microscopy and Raman spectroscopy, highlighting this compound's versatility in creating functional nanomaterials for various technological applications (Hwang et al., 2006).
Synthesis of Novel Bisterpyridines
The development of a new protocol for synthesizing novel bisterpyridine derivatives via palladium-catalyzed Miyaura- and Suzuki-type cross-couplings has expanded the utility of this compound in chemical synthesis. This efficient, mild, and broadly applicable method allows for the versatile construction of bisterpyridines, enabling symmetric and unsymmetric introduction of various substituents, thereby facilitating the creation of complex molecules for research and industrial applications (Han et al., 2007).
Bistable Systems for Energy Harvesting
Investigations into bistable energy harvesting devices have identified this compound-related materials as promising due to their unique snap-through action and nonlinear characteristics. These materials facilitate large amplitude motion, significantly enhancing power generation across a broad-frequency bandwidth, which is crucial for the efficient conversion of vibrational energy into electrical power. The exploration of bistable electromechanical dynamics with this compound-related materials underscores their potential in sustainable energy technologies (Harne & Wang, 2013).
properties
IUPAC Name |
4-(2,6-dipyridin-2-ylpyridin-4-yl)-2,6-dipyridin-2-ylpyridine | |
---|---|---|
Source | PubChem | |
URL | https://pubchem.ncbi.nlm.nih.gov | |
Description | Data deposited in or computed by PubChem | |
InChI |
InChI=1S/C30H20N6/c1-5-13-31-23(9-1)27-17-21(18-28(35-27)24-10-2-6-14-32-24)22-19-29(25-11-3-7-15-33-25)36-30(20-22)26-12-4-8-16-34-26/h1-20H | |
Source | PubChem | |
URL | https://pubchem.ncbi.nlm.nih.gov | |
Description | Data deposited in or computed by PubChem | |
InChI Key |
MJIFJDSQZXDTOT-UHFFFAOYSA-N | |
Source | PubChem | |
URL | https://pubchem.ncbi.nlm.nih.gov | |
Description | Data deposited in or computed by PubChem | |
Canonical SMILES |
C1=CC=NC(=C1)C2=CC(=CC(=N2)C3=CC=CC=N3)C4=CC(=NC(=C4)C5=CC=CC=N5)C6=CC=CC=N6 | |
Source | PubChem | |
URL | https://pubchem.ncbi.nlm.nih.gov | |
Description | Data deposited in or computed by PubChem | |
Molecular Formula |
C30H20N6 | |
Source | PubChem | |
URL | https://pubchem.ncbi.nlm.nih.gov | |
Description | Data deposited in or computed by PubChem | |
DSSTOX Substance ID |
DTXSID90475142 | |
Record name | 6',6''-Di(pyridin-2-yl)-2,2':4',4'':2'',2'''-quaterpyridine | |
Source | EPA DSSTox | |
URL | https://comptox.epa.gov/dashboard/DTXSID90475142 | |
Description | DSSTox provides a high quality public chemistry resource for supporting improved predictive toxicology. | |
Molecular Weight |
464.5 g/mol | |
Source | PubChem | |
URL | https://pubchem.ncbi.nlm.nih.gov | |
Description | Data deposited in or computed by PubChem | |
CAS RN |
128143-86-2 | |
Record name | 6',6''-Di(pyridin-2-yl)-2,2':4',4'':2'',2'''-quaterpyridine | |
Source | EPA DSSTox | |
URL | https://comptox.epa.gov/dashboard/DTXSID90475142 | |
Description | DSSTox provides a high quality public chemistry resource for supporting improved predictive toxicology. | |
Record name | 6',6''-Di(pyridin-2-yl)-2,2':4',4'':2'',2'''-quaterpyridine | |
Source | European Chemicals Agency (ECHA) | |
URL | https://echa.europa.eu/information-on-chemicals | |
Description | The European Chemicals Agency (ECHA) is an agency of the European Union which is the driving force among regulatory authorities in implementing the EU's groundbreaking chemicals legislation for the benefit of human health and the environment as well as for innovation and competitiveness. | |
Explanation | Use of the information, documents and data from the ECHA website is subject to the terms and conditions of this Legal Notice, and subject to other binding limitations provided for under applicable law, the information, documents and data made available on the ECHA website may be reproduced, distributed and/or used, totally or in part, for non-commercial purposes provided that ECHA is acknowledged as the source: "Source: European Chemicals Agency, http://echa.europa.eu/". Such acknowledgement must be included in each copy of the material. ECHA permits and encourages organisations and individuals to create links to the ECHA website under the following cumulative conditions: Links can only be made to webpages that provide a link to the Legal Notice page. | |
Retrosynthesis Analysis
AI-Powered Synthesis Planning: Our tool employs the Template_relevance Pistachio, Template_relevance Bkms_metabolic, Template_relevance Pistachio_ringbreaker, Template_relevance Reaxys, Template_relevance Reaxys_biocatalysis model, leveraging a vast database of chemical reactions to predict feasible synthetic routes.
One-Step Synthesis Focus: Specifically designed for one-step synthesis, it provides concise and direct routes for your target compounds, streamlining the synthesis process.
Accurate Predictions: Utilizing the extensive PISTACHIO, BKMS_METABOLIC, PISTACHIO_RINGBREAKER, REAXYS, REAXYS_BIOCATALYSIS database, our tool offers high-accuracy predictions, reflecting the latest in chemical research and data.
Strategy Settings
Precursor scoring | Relevance Heuristic |
---|---|
Min. plausibility | 0.01 |
Model | Template_relevance |
Template Set | Pistachio/Bkms_metabolic/Pistachio_ringbreaker/Reaxys/Reaxys_biocatalysis |
Top-N result to add to graph | 6 |
Feasible Synthetic Routes
Disclaimer and Information on In-Vitro Research Products
Please be aware that all articles and product information presented on BenchChem are intended solely for informational purposes. The products available for purchase on BenchChem are specifically designed for in-vitro studies, which are conducted outside of living organisms. In-vitro studies, derived from the Latin term "in glass," involve experiments performed in controlled laboratory settings using cells or tissues. It is important to note that these products are not categorized as medicines or drugs, and they have not received approval from the FDA for the prevention, treatment, or cure of any medical condition, ailment, or disease. We must emphasize that any form of bodily introduction of these products into humans or animals is strictly prohibited by law. It is essential to adhere to these guidelines to ensure compliance with legal and ethical standards in research and experimentation.