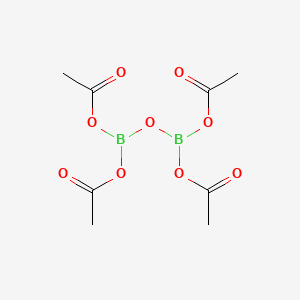
Tetraacetyl diborate
Overview
Description
Tetraacetyl diborate is an organoboron compound with the molecular formula C8H12B2O9. It is also known by its IUPAC name, [acetyloxy(diacetyloxyboranyloxy)boranyl] acetate. This compound is characterized by its colorless needle-like crystals and has a melting point of 147°C. This compound is soluble in most organic solvents and is not flammable .
Preparation Methods
Tetraacetyl diborate was discovered accidentally in the 1950s during an attempt to synthesize boron triacetate. The compound is prepared by reacting boric acid with acetic anhydride at around 75°C under a nitrogen atmosphere. This reaction produces this compound and acetic acid as by-products . The reaction can be represented as follows:
2H3BO3+5(CH3CO)2O→(CH3COO)2BOB(CH3COO)2+6CH3COOH
Chemical Reactions Analysis
Tetraacetyl diborate undergoes various chemical reactions, including substitution reactions. For instance, it reacts with methanol to form water and diacetyl methoxyboron . The reaction can be represented as:
(CH3COO)2BOB(CH3COO)2+CH3OH→(CH3COO)2BOMe+H2O
Scientific Research Applications
Tetraacetyl diborate has several applications in scientific research. It is used as a reagent in organic synthesis, particularly in the acetylation of alcohols and amines. The compound’s ability to introduce acetyl groups makes it valuable in the preparation of various organic compounds. Additionally, this compound is used in the study of boron chemistry and the development of new boron-containing materials .
Mechanism of Action
The mechanism of action of tetraacetyl diborate involves its ability to act as an acetylating agent. The compound can transfer acetyl groups to other molecules, facilitating the formation of acetylated products. This process is crucial in organic synthesis, where acetylation is a common modification used to protect functional groups or introduce new functionalities .
Comparison with Similar Compounds
Tetraacetyl diborate is unique compared to other boron-containing compounds due to its specific structure and reactivity. Similar compounds include boron triacetate and other borate esters. this compound’s ability to form stable acetylated products and its specific reaction conditions make it distinct .
Properties
IUPAC Name |
[acetyloxy(diacetyloxyboranyloxy)boranyl] acetate | |
---|---|---|
Source | PubChem | |
URL | https://pubchem.ncbi.nlm.nih.gov | |
Description | Data deposited in or computed by PubChem | |
InChI |
InChI=1S/C8H12B2O9/c1-5(11)15-9(16-6(2)12)19-10(17-7(3)13)18-8(4)14/h1-4H3 | |
Source | PubChem | |
URL | https://pubchem.ncbi.nlm.nih.gov | |
Description | Data deposited in or computed by PubChem | |
InChI Key |
ZMJHYNIVOZEJTJ-UHFFFAOYSA-N | |
Source | PubChem | |
URL | https://pubchem.ncbi.nlm.nih.gov | |
Description | Data deposited in or computed by PubChem | |
Canonical SMILES |
B(OB(OC(=O)C)OC(=O)C)(OC(=O)C)OC(=O)C | |
Source | PubChem | |
URL | https://pubchem.ncbi.nlm.nih.gov | |
Description | Data deposited in or computed by PubChem | |
Molecular Formula |
C8H12B2O9 | |
Source | PubChem | |
URL | https://pubchem.ncbi.nlm.nih.gov | |
Description | Data deposited in or computed by PubChem | |
DSSTOX Substance ID |
DTXSID80416100 | |
Record name | Tetraacetyl diborate | |
Source | EPA DSSTox | |
URL | https://comptox.epa.gov/dashboard/DTXSID80416100 | |
Description | DSSTox provides a high quality public chemistry resource for supporting improved predictive toxicology. | |
Molecular Weight |
273.8 g/mol | |
Source | PubChem | |
URL | https://pubchem.ncbi.nlm.nih.gov | |
Description | Data deposited in or computed by PubChem | |
CAS No. |
5187-37-1 | |
Record name | Tetraacetyl diborate | |
Source | EPA DSSTox | |
URL | https://comptox.epa.gov/dashboard/DTXSID80416100 | |
Description | DSSTox provides a high quality public chemistry resource for supporting improved predictive toxicology. | |
Retrosynthesis Analysis
AI-Powered Synthesis Planning: Our tool employs the Template_relevance Pistachio, Template_relevance Bkms_metabolic, Template_relevance Pistachio_ringbreaker, Template_relevance Reaxys, Template_relevance Reaxys_biocatalysis model, leveraging a vast database of chemical reactions to predict feasible synthetic routes.
One-Step Synthesis Focus: Specifically designed for one-step synthesis, it provides concise and direct routes for your target compounds, streamlining the synthesis process.
Accurate Predictions: Utilizing the extensive PISTACHIO, BKMS_METABOLIC, PISTACHIO_RINGBREAKER, REAXYS, REAXYS_BIOCATALYSIS database, our tool offers high-accuracy predictions, reflecting the latest in chemical research and data.
Strategy Settings
Precursor scoring | Relevance Heuristic |
---|---|
Min. plausibility | 0.01 |
Model | Template_relevance |
Template Set | Pistachio/Bkms_metabolic/Pistachio_ringbreaker/Reaxys/Reaxys_biocatalysis |
Top-N result to add to graph | 6 |
Feasible Synthetic Routes
Q1: How does tetraacetyl diborate act as a Lewis acid catalyst, and what advantages does it offer in organic synthesis?
A2: this compound functions as a Lewis acid catalyst due to the electron-deficient boron atoms within its structure []. It effectively catalyzes cycloaddition reactions involving unesterified acrylic acids, even under solvent-free conditions []. This is significant because it avoids the potential hazards of competing polymerization reactions that can occur with traditional Lewis acid catalysts in the presence of acrylic acids [].
Q2: Are there any specific examples of reactions where this compound has shown improved outcomes?
A3: While the provided research papers focus on the general catalytic properties of this compound, they highlight its effectiveness in promoting cycloaddition reactions with unesterified acrylic acids []. The ability to perform these reactions without solvent and with reduced polymerization risks makes this compound a potentially valuable tool in organic synthesis.
Q3: Beyond its use as a catalyst, has this compound been explored for other applications?
A4: Research on this compound has also delved into its complexation behavior. For instance, it forms a complex with sodium acetate in acetonitrile solution, resulting in the formation of the [(CH3COO)5B2O]- anion []. This finding suggests potential applications of this compound in coordination chemistry and material science.
Q4: What analytical techniques are commonly employed to characterize and study this compound?
A5: Infrared (IR) spectroscopy plays a crucial role in confirming the structure of this compound and its complexes. For example, the presence of the cyclic [(CH3COO)5B2O]- anion in the complex formed with sodium acetate is confirmed through IR spectral analysis []. This highlights the importance of spectroscopic methods in understanding the structure and properties of this compound.
Disclaimer and Information on In-Vitro Research Products
Please be aware that all articles and product information presented on BenchChem are intended solely for informational purposes. The products available for purchase on BenchChem are specifically designed for in-vitro studies, which are conducted outside of living organisms. In-vitro studies, derived from the Latin term "in glass," involve experiments performed in controlled laboratory settings using cells or tissues. It is important to note that these products are not categorized as medicines or drugs, and they have not received approval from the FDA for the prevention, treatment, or cure of any medical condition, ailment, or disease. We must emphasize that any form of bodily introduction of these products into humans or animals is strictly prohibited by law. It is essential to adhere to these guidelines to ensure compliance with legal and ethical standards in research and experimentation.