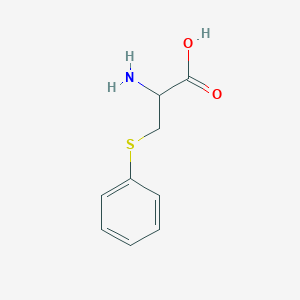
S-Phenylcysteine
- Click on QUICK INQUIRY to receive a quote from our team of experts.
- With the quality product at a COMPETITIVE price, you can focus more on your research.
Overview
Description
S-Phenylcysteine: is an organic compound that belongs to the class of amino acids It is characterized by the presence of a phenyl group attached to the sulfur atom of cysteine
Preparation Methods
Synthetic Routes and Reaction Conditions: S-Phenylcysteine can be synthesized through several methods. One efficient method involves the use of tryptophan synthase in a chemoenzymatic process. This method starts with the reaction of magnesium and bromobenzene, followed by a Grignard reaction, hydrolysis, and enzymatic synthesis using tryptophan synthase . Another method involves reacting L-cysteine hydrochloride with a soluble single-valent copper salt and the diazonium salt of phenylamine .
Industrial Production Methods: Industrial production of this compound often employs the chemoenzymatic method due to its high yield and purity. This method uses inexpensive bromobenzene and results in a total yield of 81.3% with over 99.9% purity .
Chemical Reactions Analysis
Types of Reactions: S-Phenylcysteine undergoes various chemical reactions, including oxidation, reduction, and substitution.
Common Reagents and Conditions:
Oxidation: this compound can be oxidized using reagents like hydrogen peroxide or potassium permanganate.
Reduction: Reduction reactions can be carried out using reducing agents such as sodium borohydride.
Substitution: Substitution reactions often involve nucleophiles like thiols or amines.
Major Products: The major products formed from these reactions depend on the specific conditions and reagents used. For example, oxidation can lead to the formation of sulfoxides or sulfones, while reduction can yield thiols.
Scientific Research Applications
S-Phenylcysteine has a wide range of applications in scientific research:
Chemistry: It is used as a building block in the synthesis of more complex molecules.
Biology: this compound serves as a biomarker for benzene exposure, as it forms adducts with hemoglobin.
Industry: The compound is used in the production of various pharmaceuticals and as a reagent in chemical synthesis.
Mechanism of Action
The mechanism of action of S-Phenylcysteine involves its interaction with specific molecular targets and pathways. In the context of its use as a biomarker for benzene exposure, this compound forms adducts with hemoglobin, which can be detected and quantified . As an antiretroviral agent, it is believed to inhibit HIV protease, thereby preventing the maturation of viral particles .
Comparison with Similar Compounds
S-Benzylcysteine: Similar in structure but with a benzyl group instead of a phenyl group.
S-Methylcysteine: Contains a methyl group attached to the sulfur atom.
S-tert-Butylmercapto-L-cysteine: Features a tert-butyl group attached to the sulfur atom.
Uniqueness: S-Phenylcysteine is unique due to its phenyl group, which imparts distinct chemical properties and reactivity. This uniqueness makes it particularly useful in specific applications, such as its role as a biomarker for benzene exposure and its potential as an antiretroviral agent.
Properties
CAS No. |
5437-52-5 |
---|---|
Molecular Formula |
C9H11NO2S |
Molecular Weight |
197.26 g/mol |
IUPAC Name |
2-amino-3-phenylsulfanylpropanoic acid |
InChI |
InChI=1S/C9H11NO2S/c10-8(9(11)12)6-13-7-4-2-1-3-5-7/h1-5,8H,6,10H2,(H,11,12) |
InChI Key |
XYUBQWNJDIAEES-UHFFFAOYSA-N |
SMILES |
C1=CC=C(C=C1)SCC(C(=O)O)N |
Canonical SMILES |
C1=CC=C(C=C1)SCC(C(=O)O)N |
5437-52-5 | |
physical_description |
Off-white powder; [Sigma-Aldrich MSDS] |
sequence |
X |
Synonyms |
3-phenylcysteine beta-phenylcysteine S-phenylcysteine |
Origin of Product |
United States |
Disclaimer and Information on In-Vitro Research Products
Please be aware that all articles and product information presented on BenchChem are intended solely for informational purposes. The products available for purchase on BenchChem are specifically designed for in-vitro studies, which are conducted outside of living organisms. In-vitro studies, derived from the Latin term "in glass," involve experiments performed in controlled laboratory settings using cells or tissues. It is important to note that these products are not categorized as medicines or drugs, and they have not received approval from the FDA for the prevention, treatment, or cure of any medical condition, ailment, or disease. We must emphasize that any form of bodily introduction of these products into humans or animals is strictly prohibited by law. It is essential to adhere to these guidelines to ensure compliance with legal and ethical standards in research and experimentation.