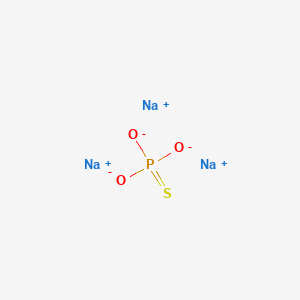
Sodium thiophosphate
- Click on QUICK INQUIRY to receive a quote from our team of experts.
- With the quality product at a COMPETITIVE price, you can focus more on your research.
Overview
Description
Sodium thiophosphate, also known as trisodium monothiophosphate, is an inorganic compound with the chemical formula Na₃PO₃S. It is a derivative of phosphoric acid where one of the oxygen atoms is replaced by a sulfur atom. This compound is known for its applications in various fields, including chemistry, biology, and industry.
Mechanism of Action
Target of Action
Sodium thiophosphate, also known as sodium phosphorothioate, primarily targets protein tyrosine phosphatase and acts as a competitive inhibitor of alkaline phosphatase . These enzymes play crucial roles in cellular processes such as cell growth, differentiation, and signal transduction .
Mode of Action
This compound interacts with its targets by forming phosphorothioate linkages in the nucleic acids of cells . This interaction makes RNA molecules more resistant to the degradative effects of nucleases within a cell, as well as during their isolation from cells .
Biochemical Pathways
This compound affects several biochemical pathways. For instance, it has been shown to improve several prebiotic reactions, including the photoreduction of thioamides, carbonyl groups, and cyanohydrins . It also enables access to tetroses and pentoses from hydrogen cyanide through a Kiliani–Fischer-type process without progressing to higher sugars . Furthermore, thiophosphate allows photochemical reductive aminations .
Pharmacokinetics
It’s known that the compound is used in various chemical reactions and can be synthesized from abundant resources via cheap and green synthesis routes .
Result of Action
The molecular and cellular effects of this compound’s action are significant. It leads to a global shift in the distribution of cellular proteins in a variety of organisms . The global changes in protein expression induced with thiophosphate could be accounted for by significant changes in the absolute transcription levels of more than 1500 genes detected via RNA seq analysis .
Action Environment
The action of this compound can be influenced by environmental factors. For instance, the phase formation of this compound can be controlled synthetically, and even subtle structural changes can significantly impact the sodium-ion diffusion in solid electrolytes . This highlights the intricate interplay between phase formation and synthetic control in the action environment of this compound .
Biochemical Analysis
Biochemical Properties
Sodium Thiophosphate participates in several biochemical reactions. It has been shown to enable prebiotic access to sugars and terpenoid precursors . This suggests that this compound could have played a role in the early stages of life on Earth. It interacts with various enzymes and proteins, enhancing certain prebiotic reactions, including the photoreduction of thioamides, carbonyl groups, and cyanohydrins .
Cellular Effects
This compound has been found to have effects on various types of cells and cellular processes. For instance, it has been shown to have a stabilizing effect towards degradation by extracellular and peroxisomal enzymes . This suggests that this compound could influence cell function by affecting enzyme activity and stability.
Molecular Mechanism
At the molecular level, this compound exerts its effects through various mechanisms. It allows photochemical reductive aminations, suggesting that it can influence the activity of enzymes and the expression of genes . Moreover, it has been shown to participate in the formation of phosphorothioate linkages in nucleic acids , which could have implications for gene expression and regulation.
Temporal Effects in Laboratory Settings
The effects of this compound can change over time in laboratory settings. For instance, it has been shown to affect the rate of reaction between a weak base and a strong acid, with the effects varying depending on temperature and concentration .
Dosage Effects in Animal Models
The effects of this compound can vary with different dosages in animal models. While specific studies on this compound are limited, it is known that the toxicity of nanoparticles, which could potentially include this compound, can affect reproduction and fetal development in female animals .
Metabolic Pathways
This compound is involved in several metabolic pathways. It has been shown to enable a plausible prebiotic synthesis of the C5 moieties used in extant terpene and terpenoid biosynthesis . This suggests that this compound could interact with enzymes and cofactors in these pathways, potentially affecting metabolic flux or metabolite levels.
Transport and Distribution
It is known that this compound can be synthesized from abundant resources via cheap and green synthesis routes, suggesting that it could be readily transported and distributed within cells .
Subcellular Localization
The localization of a protein or compound is often tied to its function . Therefore, understanding the subcellular localization of this compound could provide insights into its biochemical roles and functions.
Preparation Methods
Synthetic Routes and Reaction Conditions: Sodium thiophosphate can be synthesized through several methods. One common method involves the reaction of phosphorus pentasulfide with sodium hydroxide. The reaction proceeds as follows:
P2S5+6NaOH→2Na3PO2S2+H2S+2H2O
This reaction produces sodium dithiophosphate, which can be further hydrolyzed to form this compound.
Industrial Production Methods: In industrial settings, this compound is often produced through a solid-state reaction or a precipitation route from an aqueous solution. The solid-state reaction involves heating the reactants to high temperatures, while the precipitation route involves dissolving the reactants in water and then precipitating the product by adjusting the pH or adding a precipitating agent .
Chemical Reactions Analysis
Types of Reactions: Sodium thiophosphate undergoes various chemical reactions, including:
Oxidation: this compound can be oxidized to form sodium phosphate.
Reduction: It can be reduced to form phosphine gas.
Substitution: It can undergo substitution reactions where the sulfur atom is replaced by other atoms or groups.
Common Reagents and Conditions:
Oxidizing Agents: Hydrogen peroxide, potassium permanganate.
Reducing Agents: Lithium aluminum hydride, sodium borohydride.
Substitution Reactions: Alkyl halides, thiols.
Major Products Formed:
Oxidation: Sodium phosphate.
Reduction: Phosphine gas.
Substitution: Various organophosphorus compounds.
Scientific Research Applications
Sodium thiophosphate has a wide range of applications in scientific research:
Chemistry: It is used as a reagent in the synthesis of various organophosphorus compounds.
Biology: It is used in biochemical studies as an analogue of phosphate.
Industry: It is used in the production of flame retardants, lubricants, and as a flotation agent in mining.
Comparison with Similar Compounds
Sodium thiophosphate is similar to other thiophosphates, such as sodium dithiophosphate and sodium tetrathiophosphate. it is unique in its specific structure and reactivity:
Sodium Dithiophosphate (Na₃PO₂S₂): Contains two sulfur atoms, making it more reactive in certain substitution reactions.
Sodium Tetrathiophosphate (Na₃PS₄): Contains four sulfur atoms, which can enhance its ability to form complexes with metals.
These differences in structure lead to variations in their chemical behavior and applications .
Properties
CAS No. |
10101-88-9 |
---|---|
Molecular Formula |
H3NaO3PS |
Molecular Weight |
137.05 g/mol |
IUPAC Name |
trisodium;thiophosphate |
InChI |
InChI=1S/Na.H3O3PS/c;1-4(2,3)5/h;(H3,1,2,3,5) |
InChI Key |
XLDZOKHDPSDHMO-UHFFFAOYSA-N |
SMILES |
[O-]P(=S)([O-])[O-].[Na+].[Na+].[Na+] |
Canonical SMILES |
OP(=S)(O)O.[Na] |
10101-88-9 | |
physical_description |
Liquid |
Related CAS |
13598-51-1 (Parent) |
Synonyms |
diethyl thiophosphate sodium thiophosphate thiophosphate thiophosphoric acid thiophosphoric acid, trisodium salt |
Origin of Product |
United States |
Retrosynthesis Analysis
AI-Powered Synthesis Planning: Our tool employs the Template_relevance Pistachio, Template_relevance Bkms_metabolic, Template_relevance Pistachio_ringbreaker, Template_relevance Reaxys, Template_relevance Reaxys_biocatalysis model, leveraging a vast database of chemical reactions to predict feasible synthetic routes.
One-Step Synthesis Focus: Specifically designed for one-step synthesis, it provides concise and direct routes for your target compounds, streamlining the synthesis process.
Accurate Predictions: Utilizing the extensive PISTACHIO, BKMS_METABOLIC, PISTACHIO_RINGBREAKER, REAXYS, REAXYS_BIOCATALYSIS database, our tool offers high-accuracy predictions, reflecting the latest in chemical research and data.
Strategy Settings
Precursor scoring | Relevance Heuristic |
---|---|
Min. plausibility | 0.01 |
Model | Template_relevance |
Template Set | Pistachio/Bkms_metabolic/Pistachio_ringbreaker/Reaxys/Reaxys_biocatalysis |
Top-N result to add to graph | 6 |
Feasible Synthetic Routes
Q1: What is sodium thiophosphate (sodium phosphorothioate) and what are its primary applications in scientific research?
A1: this compound, also known as sodium phosphorothioate, is an inorganic compound with a diverse range of applications in scientific research. While not a pharmaceutical itself, its roles span from a reagent in chemical synthesis to a component in energy storage solutions and a tool in biochemical studies.
Q2: How is this compound used in the synthesis of other compounds?
A2: this compound serves as a key reagent in synthesizing various sulfur-containing compounds. For instance, it reacts with alkyl and aryl azides in aqueous solutions to produce the corresponding amines. [, ] This reaction pathway highlights its utility in introducing sulfur-containing functional groups into organic molecules.
Q3: Can you elaborate on the use of this compound in synthesizing radioprotective agents?
A3: this compound plays a crucial role in the preparation of S-2-(ω-aminoalkylamino)ethyl dihydrogen phosphorothioates, a class of compounds with potential as chemical radioprotectants. [, ] This synthesis involves reacting this compound with specific dihydrobromides in dimethylformamide, showcasing its utility in producing compounds with potential medical applications.
Q4: How does this compound contribute to the field of energy storage?
A4: this compound-based materials have gained significant attention as potential solid electrolytes for sodium-ion batteries, a promising technology for large-scale energy storage. [, , , , ] These materials, particularly in their glassy or amorphous forms, exhibit promising ionic conductivity, making them suitable for enhancing the performance and safety of solid-state batteries.
Q5: What specific advantages does this compound offer in the context of sodium-sulfur batteries?
A5: One notable advantage of this compound complexes is their ability to address a critical challenge in sodium-sulfur batteries: the formation of undesirable solid precipitates during cycling. [] These precipitates hinder battery performance by slowing down reaction kinetics and reducing cycle life. This compound complexes effectively prevent this precipitation, paving the way for more stable and efficient sodium-sulfur batteries.
Q6: Are there any drawbacks or limitations associated with the use of this compound in battery applications?
A6: While promising, this compound electrolytes face challenges related to their stability when in contact with certain electrode materials. [] These electrolytes can react with the electrodes, leading to decomposition and the formation of redox-active byproducts. This reactivity can result in excess capacity and potentially impact the long-term performance of the battery.
Q7: How is this compound employed in biochemical research?
A7: this compound serves as a valuable tool for studying enzymes, particularly phosphatases. It acts as a potent inhibitor of tyrosine phosphatases, enzymes crucial in cellular signaling pathways. [] This inhibitory action stems from the thiophosphate group's ability to compete with phosphate groups for binding sites on these enzymes.
Q8: Can you explain the mechanism behind this compound's inhibition of tyrosine phosphatases?
A8: this compound acts as a competitive inhibitor of tyrosine phosphatases by mimicking the phosphate group of natural substrates. [] The thiophosphate group binds to the enzyme's active site, preventing the binding and dephosphorylation of its intended substrates. This inhibition is specific to tyrosine phosphatases and is significantly stronger than the inhibition observed with inorganic phosphate.
Q9: What makes this compound a valuable tool in studying the structure and function of proteins?
A9: this compound enables the creation of stable analogues of phosphorylated proteins, which are otherwise challenging to study due to the lability of aspartyl phosphate bonds. [] For instance, researchers have successfully synthesized a stable analogue of the phosphorylated form of the bacterial chemotaxis protein CheB by replacing its phosphorylated aspartate residue with a cysteine residue linked to a thiophosphate group. This analogue exhibits activity comparable to the naturally phosphorylated CheB, facilitating further investigations into the protein's active form.
Q10: How does this compound contribute to the development of biosensors?
A10: this compound is utilized as a substrate for alkaline phosphatase (ALP) in the development of various biosensors. [, , , , ] In these applications, ALP catalyzes the hydrolysis of this compound to produce hydrogen sulfide (H2S). This H2S can then react with other components in the sensor system, triggering a detectable signal that correlates with the concentration of the target analyte.
Q11: Can you provide specific examples of how this compound-based biosensors work?
A11: In one example, this compound is used in a photoelectrochemical biosensor for detecting exosomes. [] ALP, linked to a detection probe, hydrolyzes this compound to generate H2S. This H2S reacts with cadmium ions to form cadmium sulfide nanoparticles, enhancing the photocurrent of the sensor and enabling the detection of exosomes.
Disclaimer and Information on In-Vitro Research Products
Please be aware that all articles and product information presented on BenchChem are intended solely for informational purposes. The products available for purchase on BenchChem are specifically designed for in-vitro studies, which are conducted outside of living organisms. In-vitro studies, derived from the Latin term "in glass," involve experiments performed in controlled laboratory settings using cells or tissues. It is important to note that these products are not categorized as medicines or drugs, and they have not received approval from the FDA for the prevention, treatment, or cure of any medical condition, ailment, or disease. We must emphasize that any form of bodily introduction of these products into humans or animals is strictly prohibited by law. It is essential to adhere to these guidelines to ensure compliance with legal and ethical standards in research and experimentation.