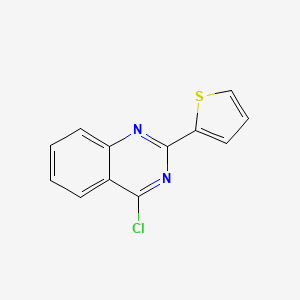
4-Chloro-2-(thiophen-2-yl)quinazoline
Overview
Description
4-Chloro-2-(thiophen-2-yl)quinazoline is a useful research compound. Its molecular formula is C12H7ClN2S and its molecular weight is 246.72 g/mol. The purity is usually 95%.
BenchChem offers high-quality this compound suitable for many research applications. Different packaging options are available to accommodate customers' requirements. Please inquire for more information about this compound including the price, delivery time, and more detailed information at info@benchchem.com.
Mechanism of Action
Target of Action
Similar quinazoline analogs have been shown to act as gaba a receptor agonists .
Mode of Action
Related quinazoline analogs have been found to provide protection against ptz-induced seizures, suggesting they may enhance the inhibitory effects of gaba neurotransmission .
Biochemical Pathways
Given its potential role as a gaba a receptor agonist, it may influence pathways related to neuronal excitability and seizure activity .
Result of Action
Related quinazoline analogs have been shown to provide protection against ptz-induced seizures, suggesting a potential anticonvulsant effect .
Biochemical Analysis
Biochemical Properties
4-Chloro-2-(thiophen-2-yl)quinazoline plays a significant role in various biochemical reactions. It interacts with several enzymes, proteins, and other biomolecules. For instance, it has been shown to inhibit the activity of α-glucosidase, an enzyme involved in carbohydrate metabolism . This inhibition is achieved through binding interactions with the enzyme’s active site, leading to a decrease in its catalytic activity. Additionally, this compound has been reported to interact with certain proteins involved in cell signaling pathways, thereby modulating their activity and influencing cellular responses .
Cellular Effects
The effects of this compound on various cell types and cellular processes are profound. It has been observed to influence cell function by altering cell signaling pathways, gene expression, and cellular metabolism. For example, in cancer cells, this compound has been shown to inhibit cell proliferation and induce apoptosis by modulating the expression of genes involved in these processes . Furthermore, it affects cellular metabolism by inhibiting key metabolic enzymes, leading to a reduction in the production of essential metabolites .
Molecular Mechanism
The molecular mechanism of action of this compound involves several key interactions at the molecular level. It binds to specific biomolecules, such as enzymes and receptors, thereby modulating their activity. For instance, it inhibits enzyme activity by binding to the active site and preventing substrate binding . Additionally, this compound can activate or inhibit signaling pathways by interacting with receptors and other signaling molecules, leading to changes in gene expression and cellular responses .
Temporal Effects in Laboratory Settings
In laboratory settings, the effects of this compound have been observed to change over time. The compound’s stability and degradation play a crucial role in its long-term effects on cellular function. Studies have shown that this compound remains stable under specific conditions, but its activity may decrease over time due to degradation . Long-term exposure to the compound has been associated with sustained inhibition of cellular processes, leading to prolonged effects on cell function .
Dosage Effects in Animal Models
The effects of this compound vary with different dosages in animal models. At lower doses, the compound exhibits therapeutic effects, such as inhibition of tumor growth and reduction of inflammation . At higher doses, it may cause toxic or adverse effects, including hepatotoxicity and nephrotoxicity . Threshold effects have been observed, where the compound’s efficacy increases with dosage up to a certain point, beyond which adverse effects become more pronounced .
Metabolic Pathways
This compound is involved in several metabolic pathways. It interacts with enzymes and cofactors that play a role in its metabolism and clearance from the body. For example, it is metabolized by cytochrome P450 enzymes, leading to the formation of metabolites that are subsequently excreted . The compound’s effects on metabolic flux and metabolite levels have been studied, revealing alterations in the levels of key metabolites involved in cellular processes .
Transport and Distribution
The transport and distribution of this compound within cells and tissues are mediated by specific transporters and binding proteins. These interactions influence the compound’s localization and accumulation in different cellular compartments . For instance, it has been shown to be transported into cells via specific membrane transporters, leading to its accumulation in the cytoplasm and other organelles . This distribution pattern affects the compound’s activity and function within the cell .
Subcellular Localization
The subcellular localization of this compound plays a crucial role in its activity and function. The compound is directed to specific compartments or organelles through targeting signals and post-translational modifications . For example, it may be localized to the nucleus, where it can interact with DNA and modulate gene expression . Alternatively, it may be targeted to the mitochondria, affecting cellular respiration and energy production .
Properties
IUPAC Name |
4-chloro-2-thiophen-2-ylquinazoline | |
---|---|---|
Source | PubChem | |
URL | https://pubchem.ncbi.nlm.nih.gov | |
Description | Data deposited in or computed by PubChem | |
InChI |
InChI=1S/C12H7ClN2S/c13-11-8-4-1-2-5-9(8)14-12(15-11)10-6-3-7-16-10/h1-7H | |
Source | PubChem | |
URL | https://pubchem.ncbi.nlm.nih.gov | |
Description | Data deposited in or computed by PubChem | |
InChI Key |
XBTCAVUUTNKLJG-UHFFFAOYSA-N | |
Source | PubChem | |
URL | https://pubchem.ncbi.nlm.nih.gov | |
Description | Data deposited in or computed by PubChem | |
Canonical SMILES |
C1=CC=C2C(=C1)C(=NC(=N2)C3=CC=CS3)Cl | |
Source | PubChem | |
URL | https://pubchem.ncbi.nlm.nih.gov | |
Description | Data deposited in or computed by PubChem | |
Molecular Formula |
C12H7ClN2S | |
Source | PubChem | |
URL | https://pubchem.ncbi.nlm.nih.gov | |
Description | Data deposited in or computed by PubChem | |
DSSTOX Substance ID |
DTXSID30366500 | |
Record name | 4-Chloro-2-(thiophen-2-yl)quinazoline | |
Source | EPA DSSTox | |
URL | https://comptox.epa.gov/dashboard/DTXSID30366500 | |
Description | DSSTox provides a high quality public chemistry resource for supporting improved predictive toxicology. | |
Molecular Weight |
246.72 g/mol | |
Source | PubChem | |
URL | https://pubchem.ncbi.nlm.nih.gov | |
Description | Data deposited in or computed by PubChem | |
CAS No. |
59455-95-7 | |
Record name | 4-Chloro-2-(thiophen-2-yl)quinazoline | |
Source | EPA DSSTox | |
URL | https://comptox.epa.gov/dashboard/DTXSID30366500 | |
Description | DSSTox provides a high quality public chemistry resource for supporting improved predictive toxicology. | |
Record name | 4-chloro-2-(thiophen-2-yl)quinazoline | |
Source | European Chemicals Agency (ECHA) | |
URL | https://echa.europa.eu/information-on-chemicals | |
Description | The European Chemicals Agency (ECHA) is an agency of the European Union which is the driving force among regulatory authorities in implementing the EU's groundbreaking chemicals legislation for the benefit of human health and the environment as well as for innovation and competitiveness. | |
Explanation | Use of the information, documents and data from the ECHA website is subject to the terms and conditions of this Legal Notice, and subject to other binding limitations provided for under applicable law, the information, documents and data made available on the ECHA website may be reproduced, distributed and/or used, totally or in part, for non-commercial purposes provided that ECHA is acknowledged as the source: "Source: European Chemicals Agency, http://echa.europa.eu/". Such acknowledgement must be included in each copy of the material. ECHA permits and encourages organisations and individuals to create links to the ECHA website under the following cumulative conditions: Links can only be made to webpages that provide a link to the Legal Notice page. | |
Synthesis routes and methods
Procedure details
Retrosynthesis Analysis
AI-Powered Synthesis Planning: Our tool employs the Template_relevance Pistachio, Template_relevance Bkms_metabolic, Template_relevance Pistachio_ringbreaker, Template_relevance Reaxys, Template_relevance Reaxys_biocatalysis model, leveraging a vast database of chemical reactions to predict feasible synthetic routes.
One-Step Synthesis Focus: Specifically designed for one-step synthesis, it provides concise and direct routes for your target compounds, streamlining the synthesis process.
Accurate Predictions: Utilizing the extensive PISTACHIO, BKMS_METABOLIC, PISTACHIO_RINGBREAKER, REAXYS, REAXYS_BIOCATALYSIS database, our tool offers high-accuracy predictions, reflecting the latest in chemical research and data.
Strategy Settings
Precursor scoring | Relevance Heuristic |
---|---|
Min. plausibility | 0.01 |
Model | Template_relevance |
Template Set | Pistachio/Bkms_metabolic/Pistachio_ringbreaker/Reaxys/Reaxys_biocatalysis |
Top-N result to add to graph | 6 |
Feasible Synthetic Routes
Disclaimer and Information on In-Vitro Research Products
Please be aware that all articles and product information presented on BenchChem are intended solely for informational purposes. The products available for purchase on BenchChem are specifically designed for in-vitro studies, which are conducted outside of living organisms. In-vitro studies, derived from the Latin term "in glass," involve experiments performed in controlled laboratory settings using cells or tissues. It is important to note that these products are not categorized as medicines or drugs, and they have not received approval from the FDA for the prevention, treatment, or cure of any medical condition, ailment, or disease. We must emphasize that any form of bodily introduction of these products into humans or animals is strictly prohibited by law. It is essential to adhere to these guidelines to ensure compliance with legal and ethical standards in research and experimentation.