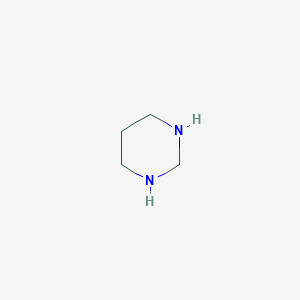
Hexahydropyrimidine
Overview
Description
Hexahydropyrimidine is a six-membered saturated heterocyclic compound containing two nitrogen atoms at positions 1 and 2. Its fully hydrogenated structure distinguishes it from aromatic pyrimidine, conferring unique conformational flexibility and reactivity. This compound derivatives are pivotal in pharmaceuticals, materials science, and energetic chemistry due to their:
- Pharmacological versatility: Anti-inflammatory, antifungal, and anticancer activities .
- Material applications: Use as polymer stabilizers, ligands for metal complexes, and precursors for energetic compounds .
- Synthetic adaptability: Synthesized via Biginelli reactions, Mannich condensations, and catalytic cycloadditions .
Preparation Methods
Hexahydropyrimidine can be synthesized through several methods, with the most common being the condensation of aldehydes or ketones with 1,3-diaminopropanes . Other methods include:
Biginelli Reaction: This involves the reaction of substituted propane-1,3-diamines with aldehydes and ketones.
Mannich Condensation: This method uses aldehydes and primary amines.
Multicomponent Reactions: These reactions involve the use of aldehydes, 1,3-dicarbonyl compounds, and urea or thiourea in the presence of various catalysts.
Industrial production methods often employ catalytic cycloaddition pathways from alkenes, which offer a more efficient and environmentally friendly approach .
Chemical Reactions Analysis
Mannich-Type Reactions
Three hexahydropyrimidine derivatives (1a–1c ) were synthesized via Mannich-type reactions using nitro-substituted aromatic aldehydes and amines. Yields varied significantly based on substituent positions:
-
5-Nitro-2,4,6-tris(4-nitrophenyl)this compound : 78% yield
-
5-Nitro-2,4,6-tris(2-nitrophenyl)this compound : 65% yield
-
5-Nitro-2,4,6-tris(3-nitrophenyl)this compound : 72% yield
Products were characterized by FT-IR, -NMR, and LC-MS .
Fe(OTf)3_33-Catalyzed Tandem Reactions
Fe(OTf) catalyzes the amidomethylation of α-phenylstyrene (B ) with bis(tosylamido)methane (A ), forming this compound (P ) in 69% yield. Key steps include:
-
Aza-Prins cyclization of allylamide intermediates.
Reduction and Substitution
Reduction of nitro-substituted hexahydropyrimidines (1a–1c ) with hydrazine yielded amino derivatives (2a–2c ). Subsequent reactions produced:
-
Schiff bases (3a–3g ): Synthesized via condensation with benzaldehyde derivatives (yields: 70–88%).
-
Amides (4a–4d ): Formed by reacting secondary amines with benzoyl chloride (yields: 68–82%) .
Nitro and Explosophore Functionalization
Twelve 1,3-dinitrothis compound derivatives were designed by introducing explosophores (–NO, –ONO, –NF). Key findings:
-
Trigger bonds : C3(5)–N5(8) bonds were identified as the weakest (bond length: 1.47–1.49 Å).
-
Detonation velocities : Ranged from 7.2–8.9 km·s, comparable to RDX (8.9 km·s) .
Fe(OTf)3_33-Catalyzed Pathway (DFT Study)
Step | Mechanism | Energy Barrier (kcal·mol) |
---|---|---|
1 | Tosylformaldimine formation | 12.4 |
2 | Intermolecular aza-Prins | 18.7 |
3 | Intramolecular cyclization | 14.2 |
The optimal pathway involves condensation → iminium homologation → intramolecular aza-Prins , validated by DFT calculations .
Hydrolysis and Reversibility
Hydrolysis of P produces 1,3-diamide 2-INT7 (52% yield), which reversibly regenerates P under acidic conditions (83% yield) .
Scientific Research Applications
Synthesis of Hexahydropyrimidine Derivatives
Hexahydropyrimidines can be synthesized through various methods, including Mannich-type reactions and reductive amination. These synthetic pathways have led to the development of numerous derivatives that exhibit a range of biological activities.
- Mannich-Type Reactions : Recent studies have successfully synthesized new this compound derivatives through this method, demonstrating noteworthy biological activities relevant to clinical applications .
- Reductive Amination : This method allows for the conversion of linear precursors into cyclic hexahydropyrimidines, facilitating access to compounds with pharmaceutical significance .
Biological Activities
This compound derivatives have been extensively studied for their biological properties, particularly in the fields of antifungal and antiviral research.
Antifungal Activity
Research has demonstrated that this compound derivatives possess significant antifungal properties against various pathogens responsible for dermatomycosis, such as Microsporum canis and Trichophyton mentagrophytes.
- A study evaluated the antifungal activity of four specific this compound derivatives, showing promising results in inhibiting fungal growth .
- The morphological changes in fungi treated with these compounds were observed using scanning electron microscopy, indicating their potential as effective antifungal agents .
Antiviral Activity
Hexahydropyrimidines have also been investigated for their antiviral properties, particularly against the Hepatitis C virus (HCV).
- A study identified a this compound core compound that inhibited HCV entry and release without affecting RNA replication, showcasing its potential as a therapeutic agent against viral infections .
Case Studies
Several case studies highlight the practical applications of this compound derivatives in real-world scenarios.
Antifungal Efficacy
In a controlled study involving cats infected with Microsporum canis, the antifungal efficacy of this compound derivatives was evaluated alongside conventional treatments like terbinafine. The results indicated that these compounds could serve as effective alternatives or adjuncts to existing antifungal therapies .
Pharmaceutical Development
The ability to synthesize hexahydropyrimidines efficiently has implications for drug development. For instance, the transformation of hydroxyproline into functionalized hexahydropyrimidines has opened avenues for creating peptidomimetics and other biologically active compounds . This highlights the compound's role in advancing pharmaceutical research and development.
Data Table: Summary of Biological Activities
Mechanism of Action
The mechanism of action of hexahydropyrimidine involves its interaction with various molecular targets and pathways. For example, in the Fe(III)-catalyzed synthesis, this compound is formed through a series of reactions including condensation, iminium homologation, and intramolecular aza-Prins reactions . These reactions are facilitated by the activation of bis(tosylamido)methane by Fe(OTf)3, leading to the formation of tosylformaldimine and its Fe(OTf)3-adduct .
Comparison with Similar Compounds
Structural and Functional Comparison with Similar Compounds
Hexahydropyrimidine vs. Pyrimidine
This compound vs. Dihydropyrimidinones (DHPMs)
DHPMs, synthesized via Biginelli reactions, are partially unsaturated derivatives with a ketone group. Key differences include:
- Pharmacology : DHPMs are studied for calcium channel modulation, while hexahydropyrimidines exhibit broader antifungal and anti-inflammatory profiles .
- Energetic Potential: this compound derivatives (e.g., 1,3-dinitrothis compound) outperform DHPMs in detonation velocity (up to 9.40 km s⁻¹) and pressure (40.20 GPa) due to nitro group substitutions .
Pharmacological Activities
Anti-inflammatory and Analgesic Effects
- This compound derivatives (e.g., compounds 11 , 23 , 4 ) show efficacy comparable to indomethacin (oral) and diclofenac (topical) .
- Mechanism: Likely inhibition of cyclooxygenase (COX) pathways, though exact targets require further study.
Antifungal Activity
- Derivatives inhibit dermatophytes (e.g., Trichophyton mentagrophytes) at MICs of 7.8–1000 µg/mL .
- Superior to pyrimidine derivatives due to enhanced membrane permeability from aliphatic substituents .
Performance Metrics
Compound | Detonation Velocity (km s⁻¹) | Detonation Pressure (GPa) | Impact Sensitivity (H₅₀, cm) |
---|---|---|---|
1,3-Dinitrothis compound | 8.62–9.40 | 34.88–40.20 | >34 |
RDX (Reference) | 8.75 | 34.9 | 24 |
Substituent Effects
- Electron-withdrawing groups (–NO₂, –C(NO₂)₃): Enhance heat of formation (HOF) and detonation performance but reduce thermal stability (N–NO₂ BDE: 80–120 kJ mol⁻¹) .
- –NF₂ and –ONO₂: Improve safety (H₅₀ >34 cm) while maintaining high energy output (D >8.6 km s⁻¹) .
Conformational and Crystallographic Insights
Biological Activity
Hexahydropyrimidine (HHP) is a nitrogen-containing heterocyclic compound that has garnered attention due to its diverse biological activities. This article reviews the biological activity of HHP, focusing on its antiviral, antifungal, and potential therapeutic applications based on recent research findings.
1. Antiviral Activity
HHP has been investigated for its role in inhibiting the Hepatitis C virus (HCV). A study demonstrated that HHP does not interfere with HCV RNA replication but instead inhibits the entry and release of viral particles. This was established through cell-based screening strategies that identified HHP as a promising candidate against HCV, showcasing its potential as an antiviral agent .
Table 1: Antiviral Mechanism of HHP
Mechanism | Effect on HCV |
---|---|
RNA Replication | No interference |
Viral Entry | Inhibited |
Viral Release | Inhibited |
2. Antifungal Activity
Research has highlighted the antifungal properties of various HHP derivatives against dermatophytes, which are fungi causing skin infections. A notable study evaluated four HHP derivatives against pathogens such as Trichophyton mentagrophytes, Microsporum canis, and Epidermophyton floccosum. Among these, one derivative (HHP3) exhibited the most potent antifungal activity, with minimum inhibitory concentrations (MICs) ranging from 500 to 1000 μg/mL .
Table 2: Antifungal Efficacy of HHP Derivatives
Compound | MIC (μg/mL) | Active Against |
---|---|---|
HHP1 | 500-1000 | T. mentagrophytes, M. canis |
HHP2 | 500-1000 | M. gypseum |
HHP3 | 250-500 | T. mentagrophytes, M. canis |
HHP4 | 500-1000 | E. floccosum |
3. Case Studies and Research Findings
In a recent investigation into the antifungal activity of silver nanoparticles combined with HHP derivatives, researchers found that these combinations could enhance efficacy against resistant fungal strains. The study highlighted the importance of developing new antifungal strategies in light of increasing drug resistance among dermatophytes .
Furthermore, another study focused on the synthesis of bicyclic hexahydropyrimidines from hydroxyproline units, indicating their potential use in creating peptidomimetics and other biologically relevant compounds . This transformation allows for the incorporation of HHP into larger molecular frameworks, enhancing its applicability in drug design.
4. Potential Therapeutic Applications
Beyond antiviral and antifungal activities, this compound derivatives are being explored for their broader therapeutic potential, including antimalarial properties. Novel compounds derived from HHP have shown promise in treating malaria, suggesting a versatile role for this compound in various therapeutic contexts .
Q & A
Basic Research Questions
Q. What are the most reliable synthetic routes for hexahydropyrimidine derivatives, and how do reaction conditions influence yield and purity?
- Methodological Answer : Classic methods include cyclocondensation of 1,3-diamines with aldehydes/ketones under reflux conditions . For example, condensation of 1,3-diaminopropan-2-ol with paraformaldehyde and phenols in methanol yields this compound derivatives after filtration and washing . Recent advances highlight catalytic cycloaddition pathways using alkenes, which reduce side reactions . Key factors affecting yield include stoichiometry, solvent polarity, and temperature gradients during reflux. Purity can be optimized via recrystallization in ethanol or methanol.
Q. How can spectroscopic and crystallographic techniques characterize this compound conformations?
- Methodological Answer : X-ray crystallography is critical for resolving torsional angles and ring conformations. For instance, torsional angles (e.g., C3-N2-C5-N6) in this compound derivatives vary between -58.6° to +84.44°, depending on substituents, as shown in CSD structural databases . Differential Scanning Calorimetry (DSC) complements this by identifying polymorphic transitions and thermal stability . NMR (¹H/¹³C) and IR spectroscopy are used to confirm functional groups and hydrogen bonding patterns, such as stabilization of N-H groups via intramolecular interactions .
Q. What are the primary biological or industrial applications of this compound derivatives?
- Methodological Answer : These derivatives serve as precursors for antimalarial agents (e.g., patented this compound antimalarials with structural modifications at positions 1 and 3) , polymer stabilizers (due to their resistance to oxidative degradation) , and protective groups in selective acylation reactions . Biological activity screening should follow standardized protocols like in vitro enzyme inhibition assays or cytotoxicity testing against Plasmodium falciparum strains .
Advanced Research Questions
Q. How do computational methods (e.g., DFT) validate experimental conformational data for this compound derivatives?
- Methodological Answer : Density Functional Theory (DFT) optimizes molecular geometries and calculates energy differences between conformers. For example, DFT-derived torsional angles (±68.7°) align with X-ray data (±68.70°), confirming the stability of face-to-face triazole conformations . Discrepancies >5° may indicate lattice packing effects or thermal motion, requiring variable-temperature crystallography to resolve . Computational workflows should include solvent-effect simulations (e.g., PCM models) and vibrational frequency analysis to match IR spectra .
Q. What strategies resolve contradictions in reported synthetic yields for this compound derivatives?
- Methodological Answer : Contradictions often arise from divergent reaction conditions (e.g., Fe(III)-catalyzed vs. uncatalyzed pathways) . Systematic analysis involves:
- Comparing solvent polarity (e.g., methanol vs. DMF) and catalyst loading.
- Replicating protocols with controlled humidity and inert atmospheres.
- Using HPLC or GC-MS to quantify byproducts and assess reaction efficiency .
Q. How do intramolecular hydrogen bonds influence the stability and reactivity of this compound derivatives?
- Methodological Answer : Hydrogen bonds between N-H and hydroxyl/phenolic groups stabilize chair conformations, as observed in 3-OH derivatives . Stability is quantified via DSC (melting points >200°C) and dynamic NMR to assess exchange rates in solution. Reactivity shifts (e.g., acylation selectivity) are studied using competitive experiments with acetylating agents (e.g., Ac₂O vs. AcCl), where H-bonded amines show reduced nucleophilicity .
Q. What are the challenges in designing this compound-based metal complexes, and how are they addressed?
- Methodological Answer : Challenges include ligand lability and steric hindrance from bulky substituents. Successful designs use:
- Polydentate ligands (e.g., N,N′-disubstituted derivatives) to enhance chelation with Rh(I) or Fe(III) .
- X-ray absorption spectroscopy (XAS) to monitor metal-ligand bond distances and oxidation states .
- Kinetic studies to optimize reaction times for complexation without ligand degradation .
Q. Data Analysis and Reproducibility
Q. How should researchers handle discrepancies in thermal expansion coefficients derived from variable-temperature crystallography?
- Methodological Answer : Discrepancies arise from anisotropic lattice vibrations. Solutions include:
- Averaging thermal parameters (e.g., Uₑq) across multiple datasets .
- Using software like TOPAS-Academic to model thermal motion tensors .
- Cross-validating with DSC data to correlate phase transitions with structural dilatation .
Q. What peer-review criteria ensure robustness in this compound research publications?
- Methodological Answer : Reviewers should assess:
- Reproducibility of synthetic protocols (e.g., explicit stoichiometry and purification steps) .
- Statistical validity of biological data (e.g., IC₅₀ values with confidence intervals) .
- Consistency between computational and experimental results (e.g., RMSD <0.5 Å for DFT-optimized structures) .
- Ethical reporting requires full disclosure of failed experiments and raw data deposition in repositories like Zenodo .
Q. Tables for Key Data
Properties
IUPAC Name |
1,3-diazinane | |
---|---|---|
Source | PubChem | |
URL | https://pubchem.ncbi.nlm.nih.gov | |
Description | Data deposited in or computed by PubChem | |
InChI |
InChI=1S/C4H10N2/c1-2-5-4-6-3-1/h5-6H,1-4H2 | |
Source | PubChem | |
URL | https://pubchem.ncbi.nlm.nih.gov | |
Description | Data deposited in or computed by PubChem | |
InChI Key |
DKYBVKMIZODYKL-UHFFFAOYSA-N | |
Source | PubChem | |
URL | https://pubchem.ncbi.nlm.nih.gov | |
Description | Data deposited in or computed by PubChem | |
Canonical SMILES |
C1CNCNC1 | |
Source | PubChem | |
URL | https://pubchem.ncbi.nlm.nih.gov | |
Description | Data deposited in or computed by PubChem | |
Molecular Formula |
C4H10N2 | |
Source | PubChem | |
URL | https://pubchem.ncbi.nlm.nih.gov | |
Description | Data deposited in or computed by PubChem | |
DSSTOX Substance ID |
DTXSID80364056 | |
Record name | 1,3-Diazacyclohexane | |
Source | EPA DSSTox | |
URL | https://comptox.epa.gov/dashboard/DTXSID80364056 | |
Description | DSSTox provides a high quality public chemistry resource for supporting improved predictive toxicology. | |
Molecular Weight |
86.14 g/mol | |
Source | PubChem | |
URL | https://pubchem.ncbi.nlm.nih.gov | |
Description | Data deposited in or computed by PubChem | |
CAS No. |
505-21-5 | |
Record name | 1,3-Diazacyclohexane | |
Source | EPA DSSTox | |
URL | https://comptox.epa.gov/dashboard/DTXSID80364056 | |
Description | DSSTox provides a high quality public chemistry resource for supporting improved predictive toxicology. | |
Retrosynthesis Analysis
AI-Powered Synthesis Planning: Our tool employs the Template_relevance Pistachio, Template_relevance Bkms_metabolic, Template_relevance Pistachio_ringbreaker, Template_relevance Reaxys, Template_relevance Reaxys_biocatalysis model, leveraging a vast database of chemical reactions to predict feasible synthetic routes.
One-Step Synthesis Focus: Specifically designed for one-step synthesis, it provides concise and direct routes for your target compounds, streamlining the synthesis process.
Accurate Predictions: Utilizing the extensive PISTACHIO, BKMS_METABOLIC, PISTACHIO_RINGBREAKER, REAXYS, REAXYS_BIOCATALYSIS database, our tool offers high-accuracy predictions, reflecting the latest in chemical research and data.
Strategy Settings
Precursor scoring | Relevance Heuristic |
---|---|
Min. plausibility | 0.01 |
Model | Template_relevance |
Template Set | Pistachio/Bkms_metabolic/Pistachio_ringbreaker/Reaxys/Reaxys_biocatalysis |
Top-N result to add to graph | 6 |
Feasible Synthetic Routes
Disclaimer and Information on In-Vitro Research Products
Please be aware that all articles and product information presented on BenchChem are intended solely for informational purposes. The products available for purchase on BenchChem are specifically designed for in-vitro studies, which are conducted outside of living organisms. In-vitro studies, derived from the Latin term "in glass," involve experiments performed in controlled laboratory settings using cells or tissues. It is important to note that these products are not categorized as medicines or drugs, and they have not received approval from the FDA for the prevention, treatment, or cure of any medical condition, ailment, or disease. We must emphasize that any form of bodily introduction of these products into humans or animals is strictly prohibited by law. It is essential to adhere to these guidelines to ensure compliance with legal and ethical standards in research and experimentation.