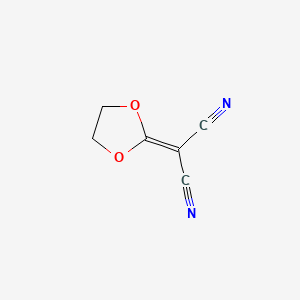
Dicyanoketene ethylene acetal
Overview
Description
Dicyanoketene ethylene acetal is an organic compound with a unique structure that includes a dioxolane ring and a dicyanomethylene group.
Preparation Methods
Dicyanoketene ethylene acetal can be synthesized through the reaction of carbonyl compounds with 1,2-ethanediol in the presence of a Brönsted or Lewis acid catalyst. The reaction typically involves the formation of a cyclic acetal, which is then further reacted with dicyanomethylene . Industrial production methods often involve the use of continuous removal of water from the reaction mixture to drive the equilibrium towards the formation of the desired product .
Chemical Reactions Analysis
Dicyanoketene ethylene acetal undergoes various chemical reactions, including:
Substitution: The compound can undergo nucleophilic substitution reactions with reagents such as organolithium or Grignard reagents.
Major Products: The major products formed from these reactions depend on the specific conditions and reagents used.
Scientific Research Applications
Dicyanoketene ethylene acetal has several scientific research applications:
Mechanism of Action
The mechanism of action of Dicyanoketene ethylene acetal involves its interaction with molecular targets through its dicyanomethylene group. This group can participate in electron transfer reactions, influencing various biochemical pathways. The compound’s effects are mediated by its ability to form stable complexes with other molecules, thereby altering their reactivity and function .
Comparison with Similar Compounds
Dicyanoketene ethylene acetal can be compared with other similar compounds such as:
1,3-Dioxane: Similar in structure but lacks the dicyanomethylene group, making it less reactive in certain applications.
5-Methyl-1,3-dioxolane-4-one: A structurally related compound used as a green solvent.
2-Methyl-1,3-dioxolane: Another related compound with different reactivity and applications.
The uniqueness of this compound lies in its combination of the dioxolane ring and the dicyanomethylene group, which imparts distinct chemical and physical properties .
Properties
IUPAC Name |
2-(1,3-dioxolan-2-ylidene)propanedinitrile | |
---|---|---|
Source | PubChem | |
URL | https://pubchem.ncbi.nlm.nih.gov | |
Description | Data deposited in or computed by PubChem | |
InChI |
InChI=1S/C6H4N2O2/c7-3-5(4-8)6-9-1-2-10-6/h1-2H2 | |
Source | PubChem | |
URL | https://pubchem.ncbi.nlm.nih.gov | |
Description | Data deposited in or computed by PubChem | |
InChI Key |
PWPZBDNODBHUSS-UHFFFAOYSA-N | |
Source | PubChem | |
URL | https://pubchem.ncbi.nlm.nih.gov | |
Description | Data deposited in or computed by PubChem | |
Canonical SMILES |
C1COC(=C(C#N)C#N)O1 | |
Source | PubChem | |
URL | https://pubchem.ncbi.nlm.nih.gov | |
Description | Data deposited in or computed by PubChem | |
Molecular Formula |
C6H4N2O2 | |
Source | PubChem | |
URL | https://pubchem.ncbi.nlm.nih.gov | |
Description | Data deposited in or computed by PubChem | |
DSSTOX Substance ID |
DTXSID90205481 | |
Record name | 1,3-Dioxolane, 2-dicyanomethylene- | |
Source | EPA DSSTox | |
URL | https://comptox.epa.gov/dashboard/DTXSID90205481 | |
Description | DSSTox provides a high quality public chemistry resource for supporting improved predictive toxicology. | |
Molecular Weight |
136.11 g/mol | |
Source | PubChem | |
URL | https://pubchem.ncbi.nlm.nih.gov | |
Description | Data deposited in or computed by PubChem | |
CAS No. |
5694-65-5 | |
Record name | 2-(1,3-Dioxolan-2-ylidene)propanedinitrile | |
Source | CAS Common Chemistry | |
URL | https://commonchemistry.cas.org/detail?cas_rn=5694-65-5 | |
Description | CAS Common Chemistry is an open community resource for accessing chemical information. Nearly 500,000 chemical substances from CAS REGISTRY cover areas of community interest, including common and frequently regulated chemicals, and those relevant to high school and undergraduate chemistry classes. This chemical information, curated by our expert scientists, is provided in alignment with our mission as a division of the American Chemical Society. | |
Explanation | The data from CAS Common Chemistry is provided under a CC-BY-NC 4.0 license, unless otherwise stated. | |
Record name | 1,3-Dioxolane, 2-dicyanomethylene- | |
Source | ChemIDplus | |
URL | https://pubchem.ncbi.nlm.nih.gov/substance/?source=chemidplus&sourceid=0005694655 | |
Description | ChemIDplus is a free, web search system that provides access to the structure and nomenclature authority files used for the identification of chemical substances cited in National Library of Medicine (NLM) databases, including the TOXNET system. | |
Record name | 1,.alpha.-malononitrile | |
Source | DTP/NCI | |
URL | https://dtp.cancer.gov/dtpstandard/servlet/dwindex?searchtype=NSC&outputformat=html&searchlist=64830 | |
Description | The NCI Development Therapeutics Program (DTP) provides services and resources to the academic and private-sector research communities worldwide to facilitate the discovery and development of new cancer therapeutic agents. | |
Explanation | Unless otherwise indicated, all text within NCI products is free of copyright and may be reused without our permission. Credit the National Cancer Institute as the source. | |
Record name | 1,3-Dioxolane, 2-dicyanomethylene- | |
Source | EPA DSSTox | |
URL | https://comptox.epa.gov/dashboard/DTXSID90205481 | |
Description | DSSTox provides a high quality public chemistry resource for supporting improved predictive toxicology. | |
Retrosynthesis Analysis
AI-Powered Synthesis Planning: Our tool employs the Template_relevance Pistachio, Template_relevance Bkms_metabolic, Template_relevance Pistachio_ringbreaker, Template_relevance Reaxys, Template_relevance Reaxys_biocatalysis model, leveraging a vast database of chemical reactions to predict feasible synthetic routes.
One-Step Synthesis Focus: Specifically designed for one-step synthesis, it provides concise and direct routes for your target compounds, streamlining the synthesis process.
Accurate Predictions: Utilizing the extensive PISTACHIO, BKMS_METABOLIC, PISTACHIO_RINGBREAKER, REAXYS, REAXYS_BIOCATALYSIS database, our tool offers high-accuracy predictions, reflecting the latest in chemical research and data.
Strategy Settings
Precursor scoring | Relevance Heuristic |
---|---|
Min. plausibility | 0.01 |
Model | Template_relevance |
Template Set | Pistachio/Bkms_metabolic/Pistachio_ringbreaker/Reaxys/Reaxys_biocatalysis |
Top-N result to add to graph | 6 |
Feasible Synthetic Routes
Disclaimer and Information on In-Vitro Research Products
Please be aware that all articles and product information presented on BenchChem are intended solely for informational purposes. The products available for purchase on BenchChem are specifically designed for in-vitro studies, which are conducted outside of living organisms. In-vitro studies, derived from the Latin term "in glass," involve experiments performed in controlled laboratory settings using cells or tissues. It is important to note that these products are not categorized as medicines or drugs, and they have not received approval from the FDA for the prevention, treatment, or cure of any medical condition, ailment, or disease. We must emphasize that any form of bodily introduction of these products into humans or animals is strictly prohibited by law. It is essential to adhere to these guidelines to ensure compliance with legal and ethical standards in research and experimentation.