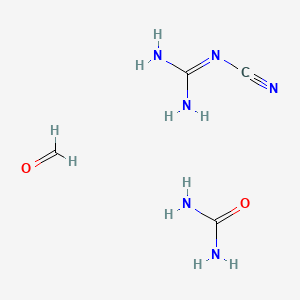
2-Cyanoguanidine;formaldehyde;urea
- Click on QUICK INQUIRY to receive a quote from our team of experts.
- With the quality product at a COMPETITIVE price, you can focus more on your research.
Overview
Description
2-Cyanoguanidine;formaldehyde;urea is a compound with the molecular formula C₄H₁₀N₆O₂. It is a polymer formed by the reaction of urea, cyanoguanidine, and formaldehyde. This compound is known for its applications in various industrial and scientific fields due to its unique chemical properties .
Preparation Methods
Synthetic Routes and Reaction Conditions
The synthesis of 2-Cyanoguanidine;formaldehyde;urea typically involves the reaction of urea, cyanoguanidine, and formaldehyde under controlled conditions. The reaction is carried out in an aqueous medium, and the pH is carefully adjusted to facilitate the polymerization process. The reaction conditions, such as temperature and time, are optimized to achieve the desired polymer structure .
Industrial Production Methods
In industrial settings, the production of this compound involves large-scale reactors where the reactants are mixed and heated to the required temperature. The reaction is monitored to ensure the formation of the polymer with the desired properties. The final product is then purified and dried for further use .
Chemical Reactions Analysis
Types of Reactions
2-Cyanoguanidine;formaldehyde;urea undergoes various chemical reactions, including:
Condensation Reactions: The compound can undergo further polymerization through condensation reactions with other aldehydes or amines.
Hydrolysis: In the presence of water and acidic or basic conditions, the polymer can hydrolyze to form its monomeric units.
Common Reagents and Conditions
Condensation Reactions: Common reagents include aldehydes and amines, with reaction conditions involving elevated temperatures and acidic or basic catalysts.
Hydrolysis: Acidic or basic conditions are used to facilitate the hydrolysis of the polymer.
Major Products Formed
The major products formed from these reactions include various derivatives of guanidine and urea, which can have applications in different fields such as agriculture and pharmaceuticals .
Scientific Research Applications
2-Cyanoguanidine;formaldehyde;urea has several scientific research applications, including:
Chemistry: Used as a precursor for the synthesis of other guanidine derivatives and polymers.
Biology: Investigated for its potential as a DNA minor groove binder and kinase inhibitor.
Medicine: Explored for its antimicrobial properties and potential use in drug development.
Mechanism of Action
The mechanism of action of 2-Cyanoguanidine;formaldehyde;urea involves its ability to form hydrogen bonds and interact with various biological molecules. The guanidine moiety in the compound is highly basic and can form stable complexes with DNA and proteins. This interaction can lead to the inhibition of certain enzymes and pathways, making it a potential candidate for drug development .
Comparison with Similar Compounds
Similar Compounds
Guanidine: A simpler compound with similar basic properties but lacks the polymeric structure of 2-Cyanoguanidine;formaldehyde;urea.
Uniqueness
This compound is unique due to its polymeric nature, which imparts distinct physical and chemical properties. Its ability to form stable complexes with various molecules makes it valuable in both industrial and scientific applications .
Properties
CAS No. |
27968-41-8 |
---|---|
Molecular Formula |
C4H10N6O2 |
Molecular Weight |
174.16 g/mol |
IUPAC Name |
2-cyanoguanidine;formaldehyde;urea |
InChI |
InChI=1S/C2H4N4.CH4N2O.CH2O/c3-1-6-2(4)5;2-1(3)4;1-2/h(H4,4,5,6);(H4,2,3,4);1H2 |
InChI Key |
ODMACUQPZFBVCI-UHFFFAOYSA-N |
SMILES |
C=O.C(#N)N=C(N)N.C(=O)(N)N |
Canonical SMILES |
C=O.C(#N)N=C(N)N.C(=O)(N)N |
Key on ui other cas no. |
27968-41-8 |
Origin of Product |
United States |
Disclaimer and Information on In-Vitro Research Products
Please be aware that all articles and product information presented on BenchChem are intended solely for informational purposes. The products available for purchase on BenchChem are specifically designed for in-vitro studies, which are conducted outside of living organisms. In-vitro studies, derived from the Latin term "in glass," involve experiments performed in controlled laboratory settings using cells or tissues. It is important to note that these products are not categorized as medicines or drugs, and they have not received approval from the FDA for the prevention, treatment, or cure of any medical condition, ailment, or disease. We must emphasize that any form of bodily introduction of these products into humans or animals is strictly prohibited by law. It is essential to adhere to these guidelines to ensure compliance with legal and ethical standards in research and experimentation.