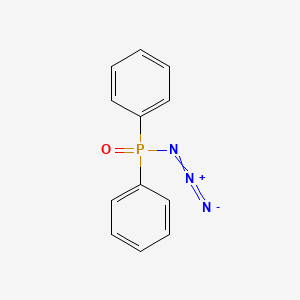
DIPHENYLPHOSPHINYL AZIDE
Overview
Description
DIPHENYLPHOSPHINYL AZIDE is an organic compound with the molecular formula C12H10N3OP. It is a colorless or faintly yellow liquid that is widely used as a reagent in organic synthesis. This compound is known for its versatility in various chemical reactions, making it a valuable tool in both academic and industrial research.
Preparation Methods
DIPHENYLPHOSPHINYL AZIDE can be synthesized through several methods. One common synthetic route involves the reaction of diphenylphosphinic chloride with sodium azide in acetone. This reaction typically yields a high amount of diphenylphosphinic azide. Another method involves the use of a quaternary ammonium salt as a phase-transfer catalyst in a biphasic system of water and an organic solvent .
Chemical Reactions Analysis
DIPHENYLPHOSPHINYL AZIDE undergoes various types of chemical reactions, including:
Substitution Reactions: It can undergo pseudohalogen replacement of the azido group by treatment with nucleophilic reagents such as ammonia and various amines.
Curtius Rearrangement: It is used to prepare acyl azides, which can then undergo the Curtius rearrangement to form isocyanates.
Reactions with Carboxylic Acids: It reacts with carboxylic acids to form urethanes or amides, depending on the reaction conditions.
Scientific Research Applications
DIPHENYLPHOSPHINYL AZIDE has a wide range of applications in scientific research:
Mechanism of Action
The mechanism of action of diphenylphosphinic azide involves the transfer of the azido group to a nucleophilic substrate. For example, in reactions with carboxylic acids, the nucleophilic carboxylate anion attacks the phosphorus atom, leading to the formation of an intermediate mixed anhydride. The azide ion is then expelled and attacks the carbonyl carbon atom, forming an acyl azide. This intermediate can further react to form urethanes or amides .
Comparison with Similar Compounds
DIPHENYLPHOSPHINYL AZIDE is similar to other azide compounds such as diphenylphosphoryl azide and diphenylphosphonic azide. it is unique in its ability to undergo a wide range of chemical reactions, making it a versatile reagent in organic synthesis. Similar compounds include:
Diphenylphosphoryl azide: Used in peptide synthesis and the Curtius rearrangement.
Diphenylphosphonic azide: Used in similar applications but with different reactivity profiles.
This compound stands out due to its high yield in synthesis and its stability under various reaction conditions .
Properties
IUPAC Name |
[azido(phenyl)phosphoryl]benzene | |
---|---|---|
Source | PubChem | |
URL | https://pubchem.ncbi.nlm.nih.gov | |
Description | Data deposited in or computed by PubChem | |
InChI |
InChI=1S/C12H10N3OP/c13-14-15-17(16,11-7-3-1-4-8-11)12-9-5-2-6-10-12/h1-10H | |
Source | PubChem | |
URL | https://pubchem.ncbi.nlm.nih.gov | |
Description | Data deposited in or computed by PubChem | |
InChI Key |
MKRTXPORKIRPDG-UHFFFAOYSA-N | |
Source | PubChem | |
URL | https://pubchem.ncbi.nlm.nih.gov | |
Description | Data deposited in or computed by PubChem | |
Canonical SMILES |
C1=CC=C(C=C1)P(=O)(C2=CC=CC=C2)N=[N+]=[N-] | |
Source | PubChem | |
URL | https://pubchem.ncbi.nlm.nih.gov | |
Description | Data deposited in or computed by PubChem | |
Molecular Formula |
C12H10N3OP | |
Source | PubChem | |
URL | https://pubchem.ncbi.nlm.nih.gov | |
Description | Data deposited in or computed by PubChem | |
DSSTOX Substance ID |
DTXSID40194275 | |
Record name | Phosphinic azide, diphenyl- | |
Source | EPA DSSTox | |
URL | https://comptox.epa.gov/dashboard/DTXSID40194275 | |
Description | DSSTox provides a high quality public chemistry resource for supporting improved predictive toxicology. | |
Molecular Weight |
243.20 g/mol | |
Source | PubChem | |
URL | https://pubchem.ncbi.nlm.nih.gov | |
Description | Data deposited in or computed by PubChem | |
CAS No. |
4129-17-3 | |
Record name | P,P-Diphenylphosphinic azide | |
Source | CAS Common Chemistry | |
URL | https://commonchemistry.cas.org/detail?cas_rn=4129-17-3 | |
Description | CAS Common Chemistry is an open community resource for accessing chemical information. Nearly 500,000 chemical substances from CAS REGISTRY cover areas of community interest, including common and frequently regulated chemicals, and those relevant to high school and undergraduate chemistry classes. This chemical information, curated by our expert scientists, is provided in alignment with our mission as a division of the American Chemical Society. | |
Explanation | The data from CAS Common Chemistry is provided under a CC-BY-NC 4.0 license, unless otherwise stated. | |
Record name | Phosphinic azide, diphenyl- | |
Source | ChemIDplus | |
URL | https://pubchem.ncbi.nlm.nih.gov/substance/?source=chemidplus&sourceid=0004129173 | |
Description | ChemIDplus is a free, web search system that provides access to the structure and nomenclature authority files used for the identification of chemical substances cited in National Library of Medicine (NLM) databases, including the TOXNET system. | |
Record name | Diphenylphosphinic azide | |
Source | DTP/NCI | |
URL | https://dtp.cancer.gov/dtpstandard/servlet/dwindex?searchtype=NSC&outputformat=html&searchlist=76046 | |
Description | The NCI Development Therapeutics Program (DTP) provides services and resources to the academic and private-sector research communities worldwide to facilitate the discovery and development of new cancer therapeutic agents. | |
Explanation | Unless otherwise indicated, all text within NCI products is free of copyright and may be reused without our permission. Credit the National Cancer Institute as the source. | |
Record name | Phosphinic azide, diphenyl- | |
Source | EPA DSSTox | |
URL | https://comptox.epa.gov/dashboard/DTXSID40194275 | |
Description | DSSTox provides a high quality public chemistry resource for supporting improved predictive toxicology. | |
Synthesis routes and methods I
Procedure details
Synthesis routes and methods II
Procedure details
Synthesis routes and methods III
Procedure details
Retrosynthesis Analysis
AI-Powered Synthesis Planning: Our tool employs the Template_relevance Pistachio, Template_relevance Bkms_metabolic, Template_relevance Pistachio_ringbreaker, Template_relevance Reaxys, Template_relevance Reaxys_biocatalysis model, leveraging a vast database of chemical reactions to predict feasible synthetic routes.
One-Step Synthesis Focus: Specifically designed for one-step synthesis, it provides concise and direct routes for your target compounds, streamlining the synthesis process.
Accurate Predictions: Utilizing the extensive PISTACHIO, BKMS_METABOLIC, PISTACHIO_RINGBREAKER, REAXYS, REAXYS_BIOCATALYSIS database, our tool offers high-accuracy predictions, reflecting the latest in chemical research and data.
Strategy Settings
Precursor scoring | Relevance Heuristic |
---|---|
Min. plausibility | 0.01 |
Model | Template_relevance |
Template Set | Pistachio/Bkms_metabolic/Pistachio_ringbreaker/Reaxys/Reaxys_biocatalysis |
Top-N result to add to graph | 6 |
Feasible Synthetic Routes
Disclaimer and Information on In-Vitro Research Products
Please be aware that all articles and product information presented on BenchChem are intended solely for informational purposes. The products available for purchase on BenchChem are specifically designed for in-vitro studies, which are conducted outside of living organisms. In-vitro studies, derived from the Latin term "in glass," involve experiments performed in controlled laboratory settings using cells or tissues. It is important to note that these products are not categorized as medicines or drugs, and they have not received approval from the FDA for the prevention, treatment, or cure of any medical condition, ailment, or disease. We must emphasize that any form of bodily introduction of these products into humans or animals is strictly prohibited by law. It is essential to adhere to these guidelines to ensure compliance with legal and ethical standards in research and experimentation.