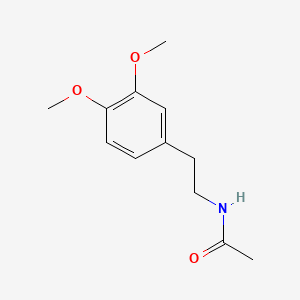
N-Acetylhomoveratrylamine
Overview
Description
N-Acetylhomoveratrylamine, also known by its IUPAC name N-[2-(3,4-dimethoxyphenyl)ethyl]acetamide, is a compound with the molecular formula C12H17NO3 It is a derivative of homoveratrylamine, characterized by the presence of an acetyl group attached to the nitrogen atom
Preparation Methods
Synthetic Routes and Reaction Conditions: N-Acetylhomoveratrylamine can be synthesized through the acetylation of β-(3,4-dimethoxyphenyl)ethylamine. The process involves adding acetic anhydride to a stirred solution of β-(3,4-dimethoxyphenyl)ethylamine in pyridine, maintaining the temperature at 90–95°C for approximately 1.5 hours . This reaction yields this compound as the primary product.
Industrial Production Methods: While specific industrial production methods for this compound are not extensively documented, the general approach involves large-scale acetylation reactions under controlled conditions to ensure high yield and purity. The use of efficient catalysts and optimized reaction parameters is crucial for industrial synthesis.
Chemical Reactions Analysis
Types of Reactions: N-Acetylhomoveratrylamine undergoes various chemical reactions, including:
Oxidation: The compound can be oxidized to form corresponding N-oxide derivatives.
Reduction: Reduction reactions can convert it back to the parent amine.
Substitution: It can participate in nucleophilic substitution reactions, particularly at the acetyl group.
Common Reagents and Conditions:
Oxidation: Common oxidizing agents include hydrogen peroxide and peracids.
Reduction: Reducing agents such as lithium aluminum hydride (LiAlH4) are typically used.
Substitution: Nucleophiles like amines or alcohols can react with this compound under basic conditions.
Major Products:
Oxidation: this compound N-oxide.
Reduction: Homoveratrylamine.
Substitution: Various substituted amides depending on the nucleophile used.
Scientific Research Applications
N-Acetylhomoveratrylamine has several applications in scientific research:
Chemistry: It serves as an intermediate in the synthesis of more complex organic molecules.
Biology: The compound is used in studies related to enzyme inhibition and receptor binding.
Medicine: Research explores its potential as a precursor for pharmaceutical compounds.
Industry: It is utilized in the production of specialty chemicals and materials.
Mechanism of Action
The mechanism by which N-Acetylhomoveratrylamine exerts its effects involves its interaction with specific molecular targets. It can act as an inhibitor or modulator of certain enzymes and receptors, influencing various biochemical pathways. The exact molecular targets and pathways depend on the specific application and context of use.
Comparison with Similar Compounds
Homoveratrylamine: The parent amine from which N-Acetylhomoveratrylamine is derived.
N-Methylhomoveratrylamine: A methylated analog with different pharmacological properties.
N-Acetylhomovanillylamine: Another acetylated derivative with distinct chemical behavior.
Uniqueness: this compound is unique due to its specific acetylation, which imparts different chemical and biological properties compared to its analogs. This uniqueness makes it valuable for targeted research and applications where specific interactions are required.
Properties
IUPAC Name |
N-[2-(3,4-dimethoxyphenyl)ethyl]acetamide | |
---|---|---|
Source | PubChem | |
URL | https://pubchem.ncbi.nlm.nih.gov | |
Description | Data deposited in or computed by PubChem | |
InChI |
InChI=1S/C12H17NO3/c1-9(14)13-7-6-10-4-5-11(15-2)12(8-10)16-3/h4-5,8H,6-7H2,1-3H3,(H,13,14) | |
Source | PubChem | |
URL | https://pubchem.ncbi.nlm.nih.gov | |
Description | Data deposited in or computed by PubChem | |
InChI Key |
WEQRLEDPPGQGOP-UHFFFAOYSA-N | |
Source | PubChem | |
URL | https://pubchem.ncbi.nlm.nih.gov | |
Description | Data deposited in or computed by PubChem | |
Canonical SMILES |
CC(=O)NCCC1=CC(=C(C=C1)OC)OC | |
Source | PubChem | |
URL | https://pubchem.ncbi.nlm.nih.gov | |
Description | Data deposited in or computed by PubChem | |
Molecular Formula |
C12H17NO3 | |
Source | PubChem | |
URL | https://pubchem.ncbi.nlm.nih.gov | |
Description | Data deposited in or computed by PubChem | |
DSSTOX Substance ID |
DTXSID70211823 | |
Record name | Acetamide, N-(3,4-dimethoxyphenethyl)- (8CI) | |
Source | EPA DSSTox | |
URL | https://comptox.epa.gov/dashboard/DTXSID70211823 | |
Description | DSSTox provides a high quality public chemistry resource for supporting improved predictive toxicology. | |
Molecular Weight |
223.27 g/mol | |
Source | PubChem | |
URL | https://pubchem.ncbi.nlm.nih.gov | |
Description | Data deposited in or computed by PubChem | |
CAS No. |
6275-29-2 | |
Record name | N-Acetyl-3,4-dimethoxyphenethylamine | |
Source | ChemIDplus | |
URL | https://pubchem.ncbi.nlm.nih.gov/substance/?source=chemidplus&sourceid=0006275292 | |
Description | ChemIDplus is a free, web search system that provides access to the structure and nomenclature authority files used for the identification of chemical substances cited in National Library of Medicine (NLM) databases, including the TOXNET system. | |
Record name | N-Acetylhomoveratrylamine | |
Source | DTP/NCI | |
URL | https://dtp.cancer.gov/dtpstandard/servlet/dwindex?searchtype=NSC&outputformat=html&searchlist=34977 | |
Description | The NCI Development Therapeutics Program (DTP) provides services and resources to the academic and private-sector research communities worldwide to facilitate the discovery and development of new cancer therapeutic agents. | |
Explanation | Unless otherwise indicated, all text within NCI products is free of copyright and may be reused without our permission. Credit the National Cancer Institute as the source. | |
Record name | N-Acetylhomoveratrylamine | |
Source | DTP/NCI | |
URL | https://dtp.cancer.gov/dtpstandard/servlet/dwindex?searchtype=NSC&outputformat=html&searchlist=33790 | |
Description | The NCI Development Therapeutics Program (DTP) provides services and resources to the academic and private-sector research communities worldwide to facilitate the discovery and development of new cancer therapeutic agents. | |
Explanation | Unless otherwise indicated, all text within NCI products is free of copyright and may be reused without our permission. Credit the National Cancer Institute as the source. | |
Record name | Acetamide, N-(3,4-dimethoxyphenethyl)- (8CI) | |
Source | EPA DSSTox | |
URL | https://comptox.epa.gov/dashboard/DTXSID70211823 | |
Description | DSSTox provides a high quality public chemistry resource for supporting improved predictive toxicology. | |
Record name | 6275-29-2 | |
Source | European Chemicals Agency (ECHA) | |
URL | https://echa.europa.eu/information-on-chemicals | |
Description | The European Chemicals Agency (ECHA) is an agency of the European Union which is the driving force among regulatory authorities in implementing the EU's groundbreaking chemicals legislation for the benefit of human health and the environment as well as for innovation and competitiveness. | |
Explanation | Use of the information, documents and data from the ECHA website is subject to the terms and conditions of this Legal Notice, and subject to other binding limitations provided for under applicable law, the information, documents and data made available on the ECHA website may be reproduced, distributed and/or used, totally or in part, for non-commercial purposes provided that ECHA is acknowledged as the source: "Source: European Chemicals Agency, http://echa.europa.eu/". Such acknowledgement must be included in each copy of the material. ECHA permits and encourages organisations and individuals to create links to the ECHA website under the following cumulative conditions: Links can only be made to webpages that provide a link to the Legal Notice page. | |
Record name | N-ACETYL-3,4-DIMETHOXYPHENETHYLAMINE | |
Source | FDA Global Substance Registration System (GSRS) | |
URL | https://gsrs.ncats.nih.gov/ginas/app/beta/substances/ZTI2KRG9EQ | |
Description | The FDA Global Substance Registration System (GSRS) enables the efficient and accurate exchange of information on what substances are in regulated products. Instead of relying on names, which vary across regulatory domains, countries, and regions, the GSRS knowledge base makes it possible for substances to be defined by standardized, scientific descriptions. | |
Explanation | Unless otherwise noted, the contents of the FDA website (www.fda.gov), both text and graphics, are not copyrighted. They are in the public domain and may be republished, reprinted and otherwise used freely by anyone without the need to obtain permission from FDA. Credit to the U.S. Food and Drug Administration as the source is appreciated but not required. | |
Synthesis routes and methods I
Procedure details
Synthesis routes and methods II
Procedure details
Synthesis routes and methods III
Procedure details
Retrosynthesis Analysis
AI-Powered Synthesis Planning: Our tool employs the Template_relevance Pistachio, Template_relevance Bkms_metabolic, Template_relevance Pistachio_ringbreaker, Template_relevance Reaxys, Template_relevance Reaxys_biocatalysis model, leveraging a vast database of chemical reactions to predict feasible synthetic routes.
One-Step Synthesis Focus: Specifically designed for one-step synthesis, it provides concise and direct routes for your target compounds, streamlining the synthesis process.
Accurate Predictions: Utilizing the extensive PISTACHIO, BKMS_METABOLIC, PISTACHIO_RINGBREAKER, REAXYS, REAXYS_BIOCATALYSIS database, our tool offers high-accuracy predictions, reflecting the latest in chemical research and data.
Strategy Settings
Precursor scoring | Relevance Heuristic |
---|---|
Min. plausibility | 0.01 |
Model | Template_relevance |
Template Set | Pistachio/Bkms_metabolic/Pistachio_ringbreaker/Reaxys/Reaxys_biocatalysis |
Top-N result to add to graph | 6 |
Feasible Synthetic Routes
Q1: What is the structural characterization of N-Acetylhomoveratrylamine?
A1: this compound, also known as N-(3,4-Dimethoxyphenethyl)acetamide, has the molecular formula C12H17NO3 and a molecular weight of 223.27 g/mol [, ]. While specific spectroscopic data isn't detailed within the provided research, this class of compounds is typically characterized using techniques like NMR (Nuclear Magnetic Resonance) and IR (Infrared) spectroscopy.
Q2: How is this compound used in the synthesis of other compounds?
A2: this compound serves as a valuable building block in organic synthesis, particularly for constructing complex heterocyclic systems. For instance, it is utilized in the synthesis of benzo[a]quinolizidines, crucial intermediates in the preparation of Ipecac alkaloids with therapeutic potential [, ]. Through Friedel-Crafts acylation reactions, this compound can be transformed into substituted 3,4-dihydro-6,7-dimethoxyisoquinolines [, ]. Further modifications of these isoquinolines can lead to pyrrolo[2,1-a]isoquinolines, benzo[a]quinolizines, and azepino[2,1-a]isoquinolines [].
Q3: Can you describe a specific synthesis pathway involving this compound?
A3: One notable synthesis pathway involves converting this compound to 2-(2-Acetamidoethyl)-4,5-dimethoxyacetophenone []. This multi-step process involves the formation of intermediates like 6,7-dimethoxy-1-methyl-3,4-dihydroisoquinoline dichlorophosphate and 2-Acetyl-6,7-dimethoxy-1-methylene-1,2,3,4-tetrahydroisoquinoline. This synthesis highlights the versatility of this compound as a starting material for diverse chemical transformations.
Q4: Has this compound been investigated for potential biological activity?
A4: While the provided research focuses primarily on synthetic applications, this compound itself has been explored as a precursor to potential reserpine analogs []. Researchers synthesized a series of amides, including N-(3-indolylethyl)-3,4-dimethoxyphenylacetamide, derived from this compound, and evaluated their ability to potentiate barbiturate hypnosis and deplete 5-hydroxytryptamine levels in rat brains. Although this particular compound exhibited limited activity compared to reserpine, this study showcases the potential of utilizing this compound as a starting point for developing biologically active molecules.
Disclaimer and Information on In-Vitro Research Products
Please be aware that all articles and product information presented on BenchChem are intended solely for informational purposes. The products available for purchase on BenchChem are specifically designed for in-vitro studies, which are conducted outside of living organisms. In-vitro studies, derived from the Latin term "in glass," involve experiments performed in controlled laboratory settings using cells or tissues. It is important to note that these products are not categorized as medicines or drugs, and they have not received approval from the FDA for the prevention, treatment, or cure of any medical condition, ailment, or disease. We must emphasize that any form of bodily introduction of these products into humans or animals is strictly prohibited by law. It is essential to adhere to these guidelines to ensure compliance with legal and ethical standards in research and experimentation.