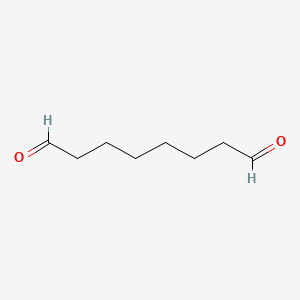
Octanedial
Overview
Description
Octanedial (CAS 765-87-9), also known as octane-1,8-dial or suberaldehyde, is a linear aliphatic dialdehyde with the molecular formula C₈H₁₄O₂. It features two terminal aldehyde groups separated by a six-carbon chain. This compound is a colorless to pale yellow liquid at room temperature, with a molecular weight of 142.20 g/mol, a boiling point of ~240–245°C, and moderate solubility in polar organic solvents such as ethanol and acetone .
This compound is primarily utilized in organic synthesis as a crosslinking agent due to its reactive aldehyde groups. It is employed in polymer chemistry to modify proteins, nucleic acids, and polysaccharides, enhancing thermal stability and mechanical properties in materials science . Its bifunctional reactivity also makes it valuable in pharmaceutical intermediates and agrochemicals.
Preparation Methods
Preparation via Epoxidation and Subsequent Oxidation
A preferred strategy for preparing 1,2-functionalized octane derivatives involves:
- Reacting 1-octene with performic acid (generated in situ from hydrogen peroxide and formic acid) to form an epoxide intermediate.
- Hydrolyzing the epoxide to a diol.
- Oxidizing the diol to the dialdehyde (octanedial).
This approach avoids expensive and toxic transition metals and uses environmentally friendly oxidants.
- Use of oxalic acid as a catalyst reduces side reactions and esterification by-products, improving purity and yield.
- Liquid-liquid extraction effectively removes oxidative impurities, minimizing odor and discoloration, crucial for high-purity products.
- The process is economically and environmentally optimized by reducing excess reactants and minimizing costly distillation steps.
Catalytic Dihydroxylation Using Transition Metals
Another documented method involves catalytic dihydroxylation of 1-octene using osmium tetroxide (OsO4) with co-catalysts and hydroperoxides, followed by oxidation:
Parameter | Details |
---|---|
Catalyst | OsO4 (0.025 g, 0.1 mmol) |
Co-catalyst | n-butyl iodide (0.037 g, 0.2 mmol) |
Oxidant | tert-butyl hydroperoxide (22 mmol) |
Solvent | tert-butyl alcohol (10.1 g) |
Temperature | 26–45 °C |
Yield | 42% selectivity to glycol (1,2-octanediol) |
This method yields 1,2-octanediol with moderate selectivity and yield but involves expensive and toxic reagents, making it less favorable for large-scale or green synthesis.
Purification and Yield Optimization
- Liquid-liquid extraction is employed to remove oxidative and esterification by-products, enhancing product purity.
- Minimizing side reactions through catalyst choice (e.g., oxalic acid) improves yield and reduces downstream purification costs.
- Avoidance or reduction of distillation steps lowers energy consumption and production costs without sacrificing purity.
Summary Table of Preparation Methods
Method | Key Reagents & Catalysts | Advantages | Disadvantages | Yield (%) |
---|---|---|---|---|
Epoxidation with performic acid + hydrolysis + oxidation | H2O2, HCOOH, oxalic acid catalyst | High purity, eco-friendly, cost-effective | Requires careful control of side reactions | High (not quantified for this compound, inferred high from diol data) |
Catalytic dihydroxylation with OsO4 + hydroperoxide | OsO4, n-butyl iodide, tert-butyl hydroperoxide | High selectivity to diol | Expensive, toxic catalyst | 42% (to diol) |
Direct oxidation (general) | Various oxidants (not detailed for this compound) | Potentially straightforward | Overoxidation risk, low selectivity | Variable |
Research Notes and Considerations
- While the literature predominantly details 1,2-octanediol synthesis, the dialdehyde this compound can be accessed by further oxidation of diol intermediates or selective cleavage of epoxides.
- The use of environmentally benign oxidants like hydrogen peroxide and formic acid is preferred over heavy metal catalysts for sustainable synthesis.
- Control of reaction pH, temperature, and catalyst loading is critical to maximize yield and purity.
- Industrial methods focus on minimizing by-products that cause odor and discoloration, essential for applications in cosmetics and household products.
Chemical Reactions Analysis
Oxidation Reactions
Octanedial undergoes oxidation to form dicarboxylic acids. Each aldehyde group () is oxidized to a carboxylic acid (), yielding suberic acid (octanedioic acid):
Conditions : Strong oxidizing agents like potassium permanganate () or chromium trioxide () in acidic media .
Reaction | Reagents/Conditions | Product |
---|---|---|
Aldehyde → Carboxylic Acid | , , heat | Suberic acid (1,8-octanedioic acid) |
Reduction Reactions
Reduction of this compound produces 1,8-octanediol , a diol with applications in polymer synthesis:
Conditions : Catalytic hydrogenation () or hydride donors () .
Reaction | Reagents/Conditions | Product |
---|---|---|
Aldehyde → Alcohol | , | 1,8-Octanediol |
Nucleophilic Addition Reactions
The electrophilic carbonyl carbons in this compound readily undergo nucleophilic additions:
Formation of Bis-Acetals
Reacting with alcohols (e.g., methanol) under acidic conditions forms cyclic or linear acetals:
Applications : Protecting aldehyde groups during multi-step syntheses .
Condensation with Amines
Primary amines (e.g., ) form bis-imines (Schiff bases):
Uses : Coordination chemistry and polymer crosslinking .
Polymerization and Crosslinking
This compound serves as a crosslinker in polymer chemistry due to its bifunctional aldehyde groups:
Reaction with Proteins
Forms stable covalent bonds with lysine residues, enabling protein immobilization or hydrogel formation:
Conditions : Mildly basic pH (8–9) .
Polycondensation with Diols
Reacts with diols (e.g., ethylene glycol) to form polyacetals:
Comparison with Related Dialdehydes
This compound’s reactivity aligns with homologous dialdehydes but varies with chain length:
Compound | Oxidation Product | Reduction Product | Key Applications |
---|---|---|---|
Glutaraldehyde | Glutaric acid | 1,5-Pentanediol | Biocides, tissue fixation |
Adipaldehyde | Adipic acid | 1,6-Hexanediol | Nylon precursor |
This compound | Suberic acid | 1,8-Octanediol | Polymers, crosslinking agents |
Thermal and Photochemical Degradation
Under elevated temperatures or UV exposure, this compound undergoes decomposition:
Scientific Research Applications
Anticalcification Treatment in Bioprosthetic Valves
One significant application of octanediol is in the treatment of glutaraldehyde-fixed bovine pericardium to prevent calcification, a common issue in bioprosthetic valves. A study evaluated the efficacy of octanediol compared to standard treatments. The results indicated that octanediol significantly reduced calcium and phosphorus content in treated samples over time.
Case Study: Efficacy of Octanedial Treatment
- Objective: Assess the anticalcification efficacy of octanediol.
- Methodology: Bovine pericardium was treated with a 5% octanediol solution and implanted in rats for 30 and 75 days.
- Results:
- At 30 days, calcium content was 0.20 mg/g for octanediol-treated samples versus 20.07 mg/g for standard treatment.
- At 75 days, calcium content was 2.36 mg/g for octanediol versus 165.61 mg/g for standard treatment.
Time (days) | Calcium Content (mg/g) | Phosphorus Content (mg/g) |
---|---|---|
30 | 0.20 (this compound) | 0.60 (this compound) |
20.07 (Standard) | 11.69 (Standard) | |
75 | 2.36 (this compound) | 1.42 (this compound) |
165.61 (Standard) | 90.90 (Standard) |
The study concluded that octanediol treatment effectively prevents calcification, suggesting its potential for enhancing the longevity of bioprosthetic valves .
Treatment for Head Louse Infestation
Another notable application of octanediol is as a novel surfactant for treating head lice infestations. Clinical studies demonstrated its effectiveness compared to traditional treatments.
Case Study: Clinical Efficacy of this compound as a Pediculicide
- Objective: Evaluate the efficacy of 1,2-octanediol lotion against head lice.
- Methodology: A multicenter study compared the effectiveness of a 20% octanediol lotion to malathion.
- Results:
- The octanediol lotion achieved a success rate of 87.9% when applied overnight compared to 47.4% for malathion.
Treatment | Success Rate (%) | Relative Risk |
---|---|---|
This compound Lotion | 87.9 | RR = 1.86 |
Malathion | 47.4 |
The findings suggest that octanediol disrupts the insect's cuticular lipid, leading to dehydration and subsequent elimination of lice .
Bioplastics Development
In material science, this compound has been explored as a plasticizer in bioplastics derived from keratin, enhancing their mechanical properties and making them suitable for food packaging and biomedical applications.
Case Study: Bioplastic from Feather Keratin
- Objective: Investigate the use of octanediol as a plasticizer for keratin films.
- Methodology: Keratin films were modified with varying concentrations of octanediol.
- Results:
- Films exhibited improved flexibility and durability compared to untreated keratin.
Property | Untreated Keratin | This compound-Plasticized Keratin |
---|---|---|
Flexibility | Low | High |
Durability | Fragile | Enhanced |
This research indicates that incorporating this compound can significantly improve the performance characteristics of bioplastics .
Bioactive Materials in Tissue Engineering
Recent studies have also explored poly(octanediol-citrate-polyglycol) copolymer in tissue engineering applications, particularly in skin wound repair.
Case Study: Skin Wound Repair with POCG
- Objective: Assess the bioactivity of POCG containing octanediol.
- Methodology: In vivo studies evaluated wound healing rates and inflammation responses.
- Results:
- POCG accelerated skin wound repair by modulating macrophage activity and promoting angiogenesis.
The study highlights the potential of this compound-based materials in enhancing tissue regeneration processes .
Mechanism of Action
The mechanism of action of octanedial involves its reactivity with nucleophiles due to the presence of two aldehyde groups. These groups can form covalent bonds with nucleophilic species, such as amines and thiols, leading to the formation of various derivatives. The molecular targets and pathways involved depend on the specific application and the nature of the reacting species.
Comparison with Similar Compounds
This section compares Octanedial with two structurally related dialdehydes: Glutaraldehyde (pentane-1,5-dial) and Adipaldehyde (hexane-1,6-dial). These compounds share functional similarities but differ in chain length, reactivity, and applications.
Structural and Physical Properties
Property | This compound (C₈H₁₄O₂) | Glutaraldehyde (C₅H₈O₂) | Adipaldehyde (C₆H₁₀O₂) |
---|---|---|---|
Molecular Weight (g/mol) | 142.20 | 100.12 | 114.14 |
Boiling Point (°C) | 240–245 | 187–189 | 210–215 |
Solubility in Water | Low | High | Moderate |
Melting Point (°C) | -20 to -15 | -14 | -30 |
Key Observations :
- Chain Length : this compound’s longer carbon chain reduces its water solubility compared to Glutaraldehyde, which is highly water-miscible due to shorter chain length and higher polarity .
- Volatility : Glutaraldehyde’s lower boiling point makes it more volatile, limiting its use in high-temperature applications compared to this compound .
Chemical Reactivity
Crosslinking Efficiency
- This compound : Exhibits slower reaction kinetics due to steric hindrance from its longer chain but provides robust crosslinking in hydrophobic matrices (e.g., polyurethane films) .
- Glutaraldehyde : Rapidly reacts with amine groups (e.g., in proteins), making it ideal for biomedical applications like tissue fixation .
- Adipaldehyde : Intermediate reactivity; used in nylon production and epoxy resins .
Stability
- This compound : More stable under acidic conditions but prone to polymerization in basic environments.
- Glutaraldehyde : Degrades rapidly in alkaline solutions, releasing toxic fumes .
Functional Contrast :
- This compound’s hydrophobicity suits it for water-resistant coatings, whereas Glutaraldehyde’s polarity favors aqueous biological systems .
- Adipaldehyde balances reactivity and stability, serving as a precursor in industrial polymer synthesis .
Research Findings and Data
Spectral Data Comparison
Technique | This compound (Key Peaks) | Glutaraldehyde (Key Peaks) |
---|---|---|
¹H NMR | δ 9.70 (CHO), 1.40–1.60 (CH₂) | δ 9.75 (CHO), 1.35–1.50 (CH₂) |
IR | 1720 cm⁻¹ (C=O stretch) | 1725 cm⁻¹ (C=O stretch) |
MS | m/z 142 (M⁺) | m/z 100 (M⁺) |
Biological Activity
Octanedial, also known as 1,2-octanediol, is a long-chain aliphatic alcohol with notable biological activities. This compound has been studied for its various applications in medicine, particularly in treating infestations and preventing calcification in biological tissues. The following sections provide an overview of its biological activity, including case studies and research findings.
This compound is characterized by its two hydroxyl groups (-OH) attached to an eight-carbon chain. Its structure allows it to interact with biological membranes, making it effective as a surfactant and antimicrobial agent. The mechanism of action primarily involves disrupting cellular membranes of pathogens, leading to cell death.
Biological Applications
-
Pediculicide (Head Lice Treatment)
- Efficacy : Clinical studies have demonstrated that 1% octanediol spray significantly reduces head lice infestations. In a randomized controlled trial involving 520 participants, the octanediol lotion showed a success rate of 87.9% after overnight application compared to 47.4% for the traditional malathion treatment .
- Safety : The treatment was generally well-tolerated, with only minor adverse effects such as transient erythema reported .
-
Anticalcification Agent
- Research Findings : A study evaluated the efficacy of octanediol in preventing calcification in glutaraldehyde-fixed bovine pericardium used in bioprosthetic valves. Results indicated that octanediol-treated samples had significantly lower calcium content (2.36 mg/g dry weight) compared to standard treatments (165.61 mg/g dry weight) after 75 days . This suggests its potential for improving the longevity and performance of bioprosthetic materials.
Case Study 1: Head Lice Infestation Treatment
- Study Design : A multicenter, randomized, observer-blind study was conducted to compare the effectiveness of 1,2-octanediol against malathion.
- Results : The study found that octanediol was significantly more effective than malathion (p<0.0005), with cure rates of 70.9% for shorter applications and 87.9% for longer applications .
Case Study 2: Anticalcification in Bioprosthetic Valves
- Objective : To assess the effectiveness of octanediol in reducing calcification in glutaraldehyde-fixed bovine pericardium.
- Findings : this compound treatment resulted in much lower calcium and phosphorus levels compared to untreated controls, indicating its efficacy in preventing calcification .
Data Table: Summary of Efficacy Studies
Study Focus | Treatment | Success Rate (%) | Adverse Effects |
---|---|---|---|
Head Lice Treatment | 1% this compound | 87.9 (overnight) | Minor erythema |
Anticalcification | This compound | Significant reduction in calcification | None reported |
Q & A
Basic Research Questions
Q. How can researchers optimize the synthesis of octanedial to improve yield and purity?
- Methodological Answer : Synthesis optimization involves systematic variation of reaction parameters (e.g., temperature, catalyst loading, solvent polarity) using Design of Experiments (DoE) frameworks. For example, kinetic studies under controlled conditions (e.g., 25–80°C) can identify rate-limiting steps, while HPLC or GC-MS analysis monitors intermediate formation . Characterization via H/C NMR and FTIR validates structural integrity, and purity is assessed via chromatographic methods (e.g., reverse-phase HPLC with UV detection) .
Q. What analytical techniques are most reliable for quantifying this compound in complex mixtures?
- Methodological Answer : Derivatization with hydrazine reagents (e.g., 2,4-dinitrophenylhydrazine) followed by LC-UV/Vis or LC-MS/MS improves selectivity in biological or environmental matrices. For real-time monitoring, headspace GC-MS minimizes matrix interference. Calibration curves must account for matrix effects, and validation should follow ICH guidelines for precision (±5% RSD) and recovery (90–110%) .
Q. How does pH influence the stability and reactivity of this compound in aqueous solutions?
- Methodological Answer : Conduct pH-dependent stability studies (pH 2–12) using buffered solutions, with aliquots sampled at intervals (0–72 hrs). Analyze degradation products via LC-HRMS and quantify residual this compound via UV spectrophotometry (λ = 260 nm). Kinetic modeling (e.g., pseudo-first-order decay) identifies dominant degradation pathways, while H NMR tracks aldehyde proton shifts under acidic/basic conditions .
Advanced Research Questions
Q. What computational methods can predict this compound’s reactivity in crosslinking reactions with biomolecules?
- Methodological Answer : Density Functional Theory (DFT) calculations (e.g., B3LYP/6-311+G(d,p)) model transition states and activation energies for Schiff base formation. Molecular dynamics (MD) simulations (AMBER force field) predict crosslinking efficiency with lysine residues in proteins. Validate predictions experimentally via MALDI-TOF MS to detect adducts and quantify reaction kinetics .
Q. How can contradictory data on this compound’s cytotoxicity be resolved across in vitro studies?
- Methodological Answer : Conduct meta-analysis of existing studies (e.g., PubMed, Scopus) to identify variables causing discrepancies (e.g., cell line heterogeneity, exposure time). Replicate key experiments under standardized conditions (e.g., ISO 10993-5 for cytotoxicity assays). Use RNA-seq to compare gene expression profiles in conflicting models and identify threshold concentrations for oxidative stress .
Q. What mechanistic insights explain this compound’s role in Maillard reaction pathways?
- Methodological Answer : Employ isotopic labeling (C-octanedial) with LC-HRMS to trace incorporation into advanced glycation end-products (AGEs). Time-resolved FTIR monitors intermediate carbonyl-amino adducts. Compare reaction rates with shorter-chain aldehydes (e.g., glyoxal) to establish structure-activity relationships. Computational docking (AutoDock Vina) predicts binding affinities to AGE receptors .
Q. How do solvent polarity and dielectric constant affect this compound’s conformational dynamics?
- Methodological Answer : Use temperature-dependent H NMR in solvents of varying polarity (e.g., DMSO, hexane). Analyze coupling constants and NOESY spectra to identify dominant conformers. MD simulations (GROMACS) correlate solvent dielectric with free energy landscapes. Compare experimental vs. computed C chemical shifts (e.g., using Gaussian 16) to validate models .
Q. Data Analysis & Experimental Design
Q. What statistical approaches are suitable for analyzing this compound’s dose-response relationships in toxicological studies?
- Methodological Answer : Apply nonlinear regression models (e.g., Hill equation) to EC50/IC50 determination. Use ANOVA with post-hoc Tukey tests for multi-group comparisons. For non-monotonic responses, Bayesian hierarchical models account for variability. Sensitivity analysis (Monte Carlo simulations) quantifies uncertainty in threshold estimates .
Q. How can researchers design reproducible assays for this compound’s oxidative stress induction?
- Methodological Answer : Standardize cell culture conditions (e.g., O levels, serum-free media) and pre-treat with antioxidants (e.g., NAC) as controls. Measure ROS via fluorescence probes (DCFH-DA) with plate readers (ex/em 485/535 nm). Include internal standards (e.g., HO) for signal normalization. Validate via Western blot for Nrf2/Keap1 pathway activation .
Properties
IUPAC Name |
octanedial | |
---|---|---|
Source | PubChem | |
URL | https://pubchem.ncbi.nlm.nih.gov | |
Description | Data deposited in or computed by PubChem | |
InChI |
InChI=1S/C8H14O2/c9-7-5-3-1-2-4-6-8-10/h7-8H,1-6H2 | |
Source | PubChem | |
URL | https://pubchem.ncbi.nlm.nih.gov | |
Description | Data deposited in or computed by PubChem | |
InChI Key |
OADYBSJSJUFUBR-UHFFFAOYSA-N | |
Source | PubChem | |
URL | https://pubchem.ncbi.nlm.nih.gov | |
Description | Data deposited in or computed by PubChem | |
Canonical SMILES |
C(CCCC=O)CCC=O | |
Source | PubChem | |
URL | https://pubchem.ncbi.nlm.nih.gov | |
Description | Data deposited in or computed by PubChem | |
Molecular Formula |
C8H14O2 | |
Source | PubChem | |
URL | https://pubchem.ncbi.nlm.nih.gov | |
Description | Data deposited in or computed by PubChem | |
DSSTOX Substance ID |
DTXSID30213247 | |
Record name | Octanedial | |
Source | EPA DSSTox | |
URL | https://comptox.epa.gov/dashboard/DTXSID30213247 | |
Description | DSSTox provides a high quality public chemistry resource for supporting improved predictive toxicology. | |
Molecular Weight |
142.20 g/mol | |
Source | PubChem | |
URL | https://pubchem.ncbi.nlm.nih.gov | |
Description | Data deposited in or computed by PubChem | |
CAS No. |
638-54-0 | |
Record name | Octanedial | |
Source | CAS Common Chemistry | |
URL | https://commonchemistry.cas.org/detail?cas_rn=638-54-0 | |
Description | CAS Common Chemistry is an open community resource for accessing chemical information. Nearly 500,000 chemical substances from CAS REGISTRY cover areas of community interest, including common and frequently regulated chemicals, and those relevant to high school and undergraduate chemistry classes. This chemical information, curated by our expert scientists, is provided in alignment with our mission as a division of the American Chemical Society. | |
Explanation | The data from CAS Common Chemistry is provided under a CC-BY-NC 4.0 license, unless otherwise stated. | |
Record name | Octanedial | |
Source | ChemIDplus | |
URL | https://pubchem.ncbi.nlm.nih.gov/substance/?source=chemidplus&sourceid=0000638540 | |
Description | ChemIDplus is a free, web search system that provides access to the structure and nomenclature authority files used for the identification of chemical substances cited in National Library of Medicine (NLM) databases, including the TOXNET system. | |
Record name | Octanedial | |
Source | EPA DSSTox | |
URL | https://comptox.epa.gov/dashboard/DTXSID30213247 | |
Description | DSSTox provides a high quality public chemistry resource for supporting improved predictive toxicology. | |
Record name | Octanedial | |
Source | European Chemicals Agency (ECHA) | |
URL | https://echa.europa.eu/substance-information/-/substanceinfo/100.010.312 | |
Description | The European Chemicals Agency (ECHA) is an agency of the European Union which is the driving force among regulatory authorities in implementing the EU's groundbreaking chemicals legislation for the benefit of human health and the environment as well as for innovation and competitiveness. | |
Explanation | Use of the information, documents and data from the ECHA website is subject to the terms and conditions of this Legal Notice, and subject to other binding limitations provided for under applicable law, the information, documents and data made available on the ECHA website may be reproduced, distributed and/or used, totally or in part, for non-commercial purposes provided that ECHA is acknowledged as the source: "Source: European Chemicals Agency, http://echa.europa.eu/". Such acknowledgement must be included in each copy of the material. ECHA permits and encourages organisations and individuals to create links to the ECHA website under the following cumulative conditions: Links can only be made to webpages that provide a link to the Legal Notice page. | |
Synthesis routes and methods I
Procedure details
Synthesis routes and methods II
Procedure details
Synthesis routes and methods III
Procedure details
Retrosynthesis Analysis
AI-Powered Synthesis Planning: Our tool employs the Template_relevance Pistachio, Template_relevance Bkms_metabolic, Template_relevance Pistachio_ringbreaker, Template_relevance Reaxys, Template_relevance Reaxys_biocatalysis model, leveraging a vast database of chemical reactions to predict feasible synthetic routes.
One-Step Synthesis Focus: Specifically designed for one-step synthesis, it provides concise and direct routes for your target compounds, streamlining the synthesis process.
Accurate Predictions: Utilizing the extensive PISTACHIO, BKMS_METABOLIC, PISTACHIO_RINGBREAKER, REAXYS, REAXYS_BIOCATALYSIS database, our tool offers high-accuracy predictions, reflecting the latest in chemical research and data.
Strategy Settings
Precursor scoring | Relevance Heuristic |
---|---|
Min. plausibility | 0.01 |
Model | Template_relevance |
Template Set | Pistachio/Bkms_metabolic/Pistachio_ringbreaker/Reaxys/Reaxys_biocatalysis |
Top-N result to add to graph | 6 |
Feasible Synthetic Routes
Disclaimer and Information on In-Vitro Research Products
Please be aware that all articles and product information presented on BenchChem are intended solely for informational purposes. The products available for purchase on BenchChem are specifically designed for in-vitro studies, which are conducted outside of living organisms. In-vitro studies, derived from the Latin term "in glass," involve experiments performed in controlled laboratory settings using cells or tissues. It is important to note that these products are not categorized as medicines or drugs, and they have not received approval from the FDA for the prevention, treatment, or cure of any medical condition, ailment, or disease. We must emphasize that any form of bodily introduction of these products into humans or animals is strictly prohibited by law. It is essential to adhere to these guidelines to ensure compliance with legal and ethical standards in research and experimentation.