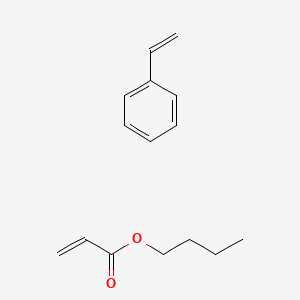
2-Propenoic acid, butyl ester, polymer with ethenylbenzene
Overview
Description
2-Propenoic acid, butyl ester, polymer with ethenylbenzene is a useful research compound. Its molecular formula is C15H20O2 and its molecular weight is 232.32 g/mol. The purity is usually 95%.
The exact mass of the compound 2-Propenoic acid, butyl ester, polymer with ethenylbenzene is unknown and the complexity rating of the compound is unknown. The storage condition is unknown. Please store according to label instructions upon receipt of goods.Use and application categories indicated by third-party sources: Cosmetics -> Film forming. However, this does not mean our product can be used or applied in the same or a similar way.
BenchChem offers high-quality 2-Propenoic acid, butyl ester, polymer with ethenylbenzene suitable for many research applications. Different packaging options are available to accommodate customers' requirements. Please inquire for more information about 2-Propenoic acid, butyl ester, polymer with ethenylbenzene including the price, delivery time, and more detailed information at info@benchchem.com.
properties
CAS RN |
25767-47-9 |
---|---|
Product Name |
2-Propenoic acid, butyl ester, polymer with ethenylbenzene |
Molecular Formula |
C15H20O2 |
Molecular Weight |
232.32 g/mol |
IUPAC Name |
butyl prop-2-enoate;styrene |
InChI |
InChI=1S/C8H8.C7H12O2/c1-2-8-6-4-3-5-7-8;1-3-5-6-9-7(8)4-2/h2-7H,1H2;4H,2-3,5-6H2,1H3 |
InChI Key |
TUZBYYLVVXPEMA-UHFFFAOYSA-N |
SMILES |
CCCCOC(=O)C=C.C=CC1=CC=CC=C1 |
Canonical SMILES |
CCCCOC(=O)C=C.C=CC1=CC=CC=C1 |
Other CAS RN |
25767-47-9 |
Origin of Product |
United States |
Synthesis routes and methods I
Procedure details
Synthesis routes and methods II
Procedure details
Synthesis routes and methods III
Procedure details
Synthesis routes and methods IV
Procedure details
Synthesis routes and methods V
Procedure details
Disclaimer and Information on In-Vitro Research Products
Please be aware that all articles and product information presented on BenchChem are intended solely for informational purposes. The products available for purchase on BenchChem are specifically designed for in-vitro studies, which are conducted outside of living organisms. In-vitro studies, derived from the Latin term "in glass," involve experiments performed in controlled laboratory settings using cells or tissues. It is important to note that these products are not categorized as medicines or drugs, and they have not received approval from the FDA for the prevention, treatment, or cure of any medical condition, ailment, or disease. We must emphasize that any form of bodily introduction of these products into humans or animals is strictly prohibited by law. It is essential to adhere to these guidelines to ensure compliance with legal and ethical standards in research and experimentation.