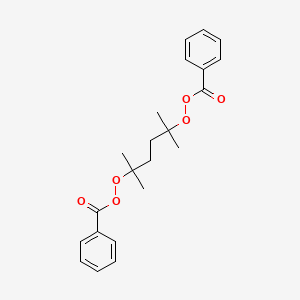
2,5-Dimethyl-2,5-di(benzoylperoxy)hexane
- Click on QUICK INQUIRY to receive a quote from our team of experts.
- With the quality product at a COMPETITIVE price, you can focus more on your research.
Description
This solid peroxide is particularly sensitive to temperature rises and contamination. Above a given "Control Temperature" they decompose violently. It is generally stored or transported as a mixture, with an inert solid.
Properties
CAS No. |
2618-77-1 |
---|---|
Molecular Formula |
C22H26O6 |
Molecular Weight |
386.4 g/mol |
IUPAC Name |
(5-benzoylperoxy-2,5-dimethylhexan-2-yl) benzenecarboperoxoate |
InChI |
InChI=1S/C22H26O6/c1-21(2,27-25-19(23)17-11-7-5-8-12-17)15-16-22(3,4)28-26-20(24)18-13-9-6-10-14-18/h5-14H,15-16H2,1-4H3 |
InChI Key |
RIPYNJLMMFGZSX-UHFFFAOYSA-N |
SMILES |
CC(C)(CCC(C)(C)OOC(=O)C1=CC=CC=C1)OOC(=O)C2=CC=CC=C2 |
Canonical SMILES |
CC(C)(CCC(C)(C)OOC(=O)C1=CC=CC=C1)OOC(=O)C2=CC=CC=C2 |
2618-77-1 | |
physical_description |
This solid peroxide is particularly sensitive to temperature rises and contamination. Above a given "Control Temperature" they decompose violently. It is generally stored or transported as a mixture, with an inert solid. |
Pictograms |
Explosive; Flammable |
Origin of Product |
United States |
Retrosynthesis Analysis
AI-Powered Synthesis Planning: Our tool employs the Template_relevance Pistachio, Template_relevance Bkms_metabolic, Template_relevance Pistachio_ringbreaker, Template_relevance Reaxys, Template_relevance Reaxys_biocatalysis model, leveraging a vast database of chemical reactions to predict feasible synthetic routes.
One-Step Synthesis Focus: Specifically designed for one-step synthesis, it provides concise and direct routes for your target compounds, streamlining the synthesis process.
Accurate Predictions: Utilizing the extensive PISTACHIO, BKMS_METABOLIC, PISTACHIO_RINGBREAKER, REAXYS, REAXYS_BIOCATALYSIS database, our tool offers high-accuracy predictions, reflecting the latest in chemical research and data.
Strategy Settings
Precursor scoring | Relevance Heuristic |
---|---|
Min. plausibility | 0.01 |
Model | Template_relevance |
Template Set | Pistachio/Bkms_metabolic/Pistachio_ringbreaker/Reaxys/Reaxys_biocatalysis |
Top-N result to add to graph | 6 |
Feasible Synthetic Routes
Disclaimer and Information on In-Vitro Research Products
Please be aware that all articles and product information presented on BenchChem are intended solely for informational purposes. The products available for purchase on BenchChem are specifically designed for in-vitro studies, which are conducted outside of living organisms. In-vitro studies, derived from the Latin term "in glass," involve experiments performed in controlled laboratory settings using cells or tissues. It is important to note that these products are not categorized as medicines or drugs, and they have not received approval from the FDA for the prevention, treatment, or cure of any medical condition, ailment, or disease. We must emphasize that any form of bodily introduction of these products into humans or animals is strictly prohibited by law. It is essential to adhere to these guidelines to ensure compliance with legal and ethical standards in research and experimentation.