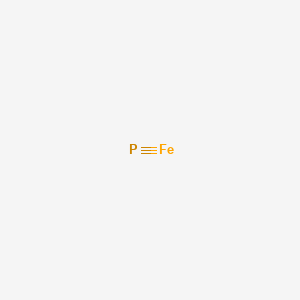
Iron phosphide (FeP)
Overview
Description
Iron phosphide (FeP) is a transition metal phosphide (TMP) with an orthorhombic crystal structure (ICDD 03-065-2595) and nano-rod morphology, synthesized via solvothermal methods . It is notable for its metallic conductivity, high theoretical capacity (e.g., 926 mAh g⁻¹ for sodium-ion batteries), and catalytic activity in energy conversion/storage applications such as the hydrogen evolution reaction (HER), supercapacitors, and alkali-metal-ion batteries . FeP’s performance is enhanced by hybridization with carbon matrices, which improve conductivity and stability . However, pure FeP surfaces are prone to oxidation, forming oxides or phosphates in air .
Preparation Methods
Solution-Based Synthesis Using Iron Oxyhydroxide and Tri-n-octylphosphine
A prominent method involves a solution-phase reaction using iron oxyhydroxide (β-FeOOH) as the iron source and tri-n-octylphosphine (TOP) as the phosphorus source and solvent. This approach is cost-effective, environmentally friendly, and allows for control over the crystalline phase of FeP.
- β-FeOOH powder (0.66 mmol, 0.059 g) is mixed with 5.38 mmol of TOP.
- The mixture is heated under an argon atmosphere at 320 °C.
- Reaction parameters such as heating rate, reaction time, and TOP amount are optimized to favor FeP formation.
- Fast heating rates (approximately 18.8 °C/min) and longer reaction times favor phosphorus-rich FeP formation.
- Slow heating rates (around 4.5 °C/min) and shorter times tend to produce mixtures of FeP and Fe2P phases.
Morphology and Characterization:
- The FeP forms nanobundles with individual rod/branch dimensions ranging from 500 nm to 1 μm.
- High-resolution transmission electron microscopy (HRTEM) reveals lattice fringes corresponding to the (020) plane of FeP with a d-spacing of 0.29 nm.
- Powder X-ray diffraction (pXRD) confirms the crystalline phase of FeP.
Parameter | Effect on FeP Formation |
---|---|
Heating Rate | Fast heating (18.8 °C/min) favors pure FeP phase |
Reaction Time | Longer times favor phosphorus-rich FeP |
TOP Amount | Sufficient TOP ensures phosphorus availability |
Temperature | 320 °C optimal for nanobundle formation |
This method yields FeP nanoparticles with excellent charge transfer kinetics and high electrical conductivity, suitable for catalytic applications.
Water-Dissolvable Template-Based Synthesis for Ultrathin FeP
This method employs a water-dissolvable template to synthesize ultrathin two-dimensional (2D) FeP nanosheets. It enables scalable production of free-standing ultrathin iron phosphide with tunable morphology.
- A water-soluble template is used to guide the formation of FeP nanosheets.
- The aging process controls the morphology, allowing transformation from mesoporous nanosheets to hierarchical nanonets.
- The process produces ultrathin FeP with high surface area and enhanced catalytic properties.
- The FeP produced exhibits a low overpotential of 117 mV and a Tafel slope of 56 mV/dec for HER.
- The 2D morphology enhances catalytic activity compared to bulk FeP materials.
- Scalability and facile control over morphology.
- Production of free-standing, ultrathin FeP materials with unique properties.
This method broadens the scope of FeP synthesis by enabling novel 2D structures with improved catalytic performance.
Comparative Summary of Preparation Methods
Method | Iron Source | Phosphorus Source | Conditions | Morphology | Advantages | Limitations |
---|---|---|---|---|---|---|
Solution-based (β-FeOOH + TOP) | β-FeOOH (iron oxyhydroxide) | Tri-n-octylphosphine (TOP) | 320 °C, Ar atmosphere, fast heating | Nanobundles (500 nm - 1 μm rods) | Cost-effective, phase control, nanostructured | Requires inert atmosphere, organic solvents |
Water-dissolvable template | Iron salts/compounds | Phosphorus source (varied) | Template-assisted, aging process | Ultrathin 2D nanosheets | Scalable, morphology tunability | Template removal step needed |
High-pressure elemental reaction | Elemental Fe powder | Red phosphorus powder | ~60 kbar, 1100 °C | Bulk crystalline phases | Access to high-P phases | Specialized equipment, less morphology control |
Research Findings and Optimization Insights
- Heating Rate: Fast heating rates promote the formation of phosphorus-rich FeP phases, while slow rates lead to mixed phases including Fe2P.
- Reaction Time: Extended reaction times enhance phosphorus incorporation and phase purity.
- Phosphorus Source: Tri-n-octylphosphine serves both as a solvent and phosphorus donor, enabling controlled phosphorus delivery.
- Morphology Control: Solution-based synthesis yields nanobundles with high surface area, beneficial for catalytic applications.
- Template Methods: Enable creation of ultrathin 2D FeP structures with unique electronic and catalytic properties.
- High-Pressure Synthesis: Useful for exploring new phosphide phases but less practical for nanostructured materials.
Chemical Reactions Analysis
Decomposition and Stability
FeP reacts with moisture and acids, producing phosphine (PH₃), a toxic and pyrophoric gas :
Surface oxidation in air generates iron phosphate species (e.g., PO₄³⁻), as confirmed by XPS .
Electrocatalytic Hydrogen Evolution (HER)
FeP nanobundles demonstrate strong HER activity due to synergistic Fe and P sites facilitating proton adsorption and H₂ release .
Table 2: HER Performance of FeP Nanobundles
Electrolyte | Overpotential (mV) | Tafel Slope (mV/dec) | Stability (hr) |
---|---|---|---|
0.5 M H₂SO₄ | 170 | 52 | 24 |
1 M KOH | 220 | 67 | 20 |
The electron density transfer from Fe to P (evidenced by XPS ) creates active sites for H⁺ adsorption (on P) and H₂ desorption (from Fe), enhancing efficiency .
Surface Oxidation and Passivation
Exposure to air forms a phosphate-rich layer (e.g., FePO₄), which passivates the surface but reduces catalytic activity. XPS confirms oxidized P species (133.8 eV binding energy) alongside intact FeP (129.5 eV) .
Key Research Findings
-
Phase Control : Fast heating rates (≥18.8°C/min) are critical for FeP synthesis, avoiding Fe₂P impurities .
-
HER Mechanism : FeP follows a Volmer-Heyrovsky pathway in acidic media, with P sites adsorbing H⁺ and Fe sites releasing H₂ .
-
Stability Limits : Prolonged HER operation (>24 hr) induces surface oxidation, necessitating protective coatings for industrial use .
Scientific Research Applications
Catalytic Applications
Hydrogen Evolution Reaction (HER)
FeP nanoparticles exhibit excellent catalytic properties for the hydrogen evolution reaction, which is crucial for sustainable energy production. Their high electrical conductivity and fast charge transfer kinetics make them promising candidates for use in electrochemical cells. A recent study demonstrated a solution-based synthesis method that optimizes the formation of FeP nanobundles, enhancing their catalytic performance for HER .
Oxygen Evolution Reaction (OER)
FeP nanorods have been synthesized through hydrothermal methods and shown to possess outstanding catalytic activity and stability for the oxygen evolution reaction. These nanorods, when dispersed on conductive substrates like carbon fiber paper, facilitate efficient water splitting processes, which are essential for renewable energy applications .
Environmental Remediation
In environmental chemistry, FeP has been explored as a novel material for the activation of persulfate in the oxidation of organic pollutants. This application highlights its potential in treating wastewater and remediating contaminated environments .
Energy Storage Applications
Lithium-Ion Batteries
FeP has emerged as a promising anode material in lithium-ion batteries due to its high theoretical capacity and favorable electrochemical properties. A comprehensive study showed that FeP exhibits a capacity retention of 53.7% over 50 cycles when used as an anode material in full-cell configurations with lithium nickel manganese cobalt oxide (Li(NiMnCo)O2) cathodes. This performance is attributed to both insertion and conversion reactions occurring during lithium intercalation .
Supercapacitors
Research indicates that FeP can also be utilized in supercapacitors, where its high surface area and conductivity contribute to enhanced charge storage capabilities. The development of flexible FeP electrodes has paved the way for lightweight and efficient energy storage devices .
Electronic Applications
Semiconductors
FeP is recognized as a semiconductor material suitable for high-power and high-frequency applications. Its unique electronic properties make it an attractive candidate for use in laser diodes and other electronic devices where efficient charge transport is essential .
Synthesis Techniques
The synthesis of iron phosphide can significantly impact its properties and applications. Various methods have been developed, including:
- Solution-Based Methods : Utilizing iron oxyhydroxide as a precursor allows for controlled synthesis of FeP nanoparticles with desired morphologies .
- Hydrothermal Synthesis : This technique enables the production of uniform FeP nanorods with enhanced catalytic performance for OER .
- Phosphorization Processes : Transforming iron oxides into FeP through phosphorization has been shown to yield materials with excellent electrochemical characteristics .
Case Studies
Mechanism of Action
The mechanism by which iron phosphide exerts its effects, particularly in electrocatalysis, involves the following:
Catalytic Sites: Iron phosphide provides active sites for the adsorption and dissociation of water molecules during the hydrogen evolution reaction.
Electronic Structure: The modification of the electronic structure of iron phosphide enhances its catalytic performance by increasing the number of accessible active sites and improving conductivity.
Synergy with Carbon: When combined with carbon materials, iron phosphide exhibits improved stability and activity due to the strong synergy between the nanoparticles and the carbon matrix.
Comparison with Similar Compounds
Comparison with Other Iron Phosphides
FeP vs. Fe₂P
- Synthesis: Fe₂P is prepared using ionic liquids (ILs) as dual Fe and P sources, yielding Fe₂P/CNTs with low HER overpotentials (75 mV onset, 115 mV for 10 mA cm⁻²) and Tafel slopes (68 mV dec⁻¹) . FeP synthesis often requires harsher conditions, such as high-temperature phosphidation (593 K) or solvothermal routes .
- Catalytic Performance : Fe₂P/CNTs show superior HER activity compared to FeP in acidic conditions. However, core-shell FeP/Fe₃O₄ heterostructures outperform pure FeP in HER due to synergistic electronic interactions, despite higher charge-transfer resistance .
- Phase Stability : FeP is more stable than Fe₂P in hydrotreating reactions; FeP/C catalysts exhibit better durability than Fe₂P/C .
FeP vs. Fe₃P and FeP₂
- Fe₃P and FeP₂ are less studied due to synthesis challenges. FeP₂, with higher P content, shows distinct electronic properties but is less explored in catalysis .
Comparison with Other Transition Metal Phosphides
MoP and WP
- MoP and WP exhibit excellent HER activity but suffer from high costs and scarcity. FeP is more economical (Fe: ~5% Earth’s crust abundance) and offers competitive performance in carbon-supported forms .
CoP and NiP
- CoP and NiP have lower HER overpotentials than FeP (e.g., CoP: ~85 mV for 10 mA cm⁻²). However, FeP’s cost-effectiveness and environmental benignity make it preferable for large-scale applications .
Structural and Electronic Differences
- FeP is metallic, whereas NiP₂ is a semiconductor. This distinction affects charge transfer in electrocatalysis .
- Crystallographic facets influence HER activity; FeP’s (31−1) and (011) planes enhance catalytic anisotropy .
Hydrogen Evolution Reaction (HER)
Compound | Onset Overpotential (mV) | Tafel Slope (mV dec⁻¹) | Stability (Cycles) | Reference |
---|---|---|---|---|
Fe₂P/CNTs | 75 | 68 | >10,000 | |
FeP/Fe₃O₄ | 90 | 72 | >5,000 | |
CoP | 85 | 65 | >8,000 |
Supercapacitors
Compound | Areal Capacitance (mF cm⁻²) | Capacitance Retention | Reference |
---|---|---|---|
FeP/C | 313 (1.2 mA cm⁻²) | 95% after 10,000 | |
P/C (Blank) | 150 | 90% |
Sodium-Ion Batteries
Compound | Capacity (mAh g⁻¹) | Cycle Stability | Reference |
---|---|---|---|
FeP@NPC Film | 926 (0.1 A g⁻¹) | 80% after 1,000 cycles |
Key Research Findings
Synthesis Innovations: IL-based methods for Fe₂P are eco-friendly and scalable, while FeP benefits from carbon hybridization to mitigate oxidation .
Phase-Dependent Activity : FeP/Fe₂P heterostructures leverage built-in electric fields to enhance catalytic and energy storage performance .
Magnetic Properties: Pure FeP is non-magnetic, distinguishing it from Fe-P mixtures containing metallic iron .
Biological Activity
Iron phosphide (FeP) is an intriguing compound that has garnered attention for its potential biological applications, particularly in catalysis and energy conversion processes. This article aims to explore the biological activity of FeP, highlighting its synthesis, properties, and applications based on recent research findings.
Synthesis of Iron Phosphide
FeP can be synthesized through various methods, including:
- Solution-Based Processes : This method involves using iron oxyhydroxide (β-FeOOH) as a precursor and tri-n-octylphosphine (TOP) as the phosphorus source. The reaction typically occurs at elevated temperatures (around 320 °C), resulting in FeP nanobundles with excellent catalytic properties .
- Electrochemical Methods : FeP has also been investigated as a catalyst in electrochemical reactions, particularly in hydrogen evolution reactions (HER). Its unique morphology and electronic structure contribute to its efficiency as an electrocatalyst .
Properties of Iron Phosphide
FeP exhibits several notable properties that enhance its biological activity:
- High Electrical Conductivity : FeP nanoparticles demonstrate fast charge transfer kinetics, which is crucial for their role in catalysis .
- Catalytic Efficiency : FeP has shown promising results as an electrocatalyst for HER, with amorphous forms outperforming crystalline counterparts in various electrolytic environments .
- Surface Characteristics : The surface morphology of FeP influences its catalytic performance. Nanostructured forms provide a higher electrochemically active surface area (ECSA), facilitating better interaction with reactants .
Electrocatalysis
Iron phosphide is primarily recognized for its role in electrocatalysis:
- Hydrogen Evolution Reaction (HER) : FeP serves as an efficient catalyst for HER, exhibiting lower overpotential values compared to other materials. Studies have shown that the active centers in FeP are structurally similar to those found in biological hydrogenase enzymes, which enhances its catalytic efficiency .
- Ammonia Synthesis : Research indicates that FeP can facilitate ammonia synthesis under electric fields, demonstrating high activity through an associative mechanism rather than direct cleavage pathways. This property could have significant implications for sustainable ammonia production .
Case Studies
- FeP Nanobundles for HER :
- Electrochemical Performance :
Data Tables
Property | Value |
---|---|
Molecular Weight | 86.82 g/mol |
Crystallographic Structure | Orthorhombic |
BET Surface Area | 4.12 m²/g |
Total Pore Volume | 0.018 cm³/g |
ECSA | 23 μF/cm² |
Q & A
Q. Basic: What are the standard synthesis methods for producing phase-pure FeP nanoparticles?
Methodological Answer:
Phase-pure FeP nanoparticles are typically synthesized via colloidal routes or solid-state reactions. A widely adopted method involves using a stoichiometric phosphorus precursor (e.g., tris(trimethylsilyl)phosphine) and iron precursors (e.g., iron carbonyls) in high-boiling solvents (e.g., octadecene). Temperature control (180–320°C) and inert atmospheres (N₂/Ar) are critical to prevent oxidation . Post-synthesis, X-ray diffraction (XRD) and transmission electron microscopy (TEM) should confirm crystallinity and phase purity, while X-ray photoelectron spectroscopy (XPS) identifies surface oxidation states .
Table 1: Key Parameters for FeP Synthesis
Parameter | Optimal Range | Purpose |
---|---|---|
Temperature | 180–320°C | Phase selectivity (amorphous vs. crystalline) |
Precursor Ratio | Fe:P = 1:1 | Stoichiometric control |
Reaction Time | 1–3 hours | Crystallization completion |
Q. Advanced: How can crystallinity and surface oxidation of FeP be controlled during synthesis to optimize electrocatalytic activity?
Methodological Answer:
Crystallinity is modulated by adjusting annealing temperatures and precursor decomposition rates. For example, annealing at 320°C promotes crystalline FeP, while lower temperatures (180°C) yield amorphous phases with higher catalytic surface area . To mitigate surface oxidation (e.g., FePO₄ formation), in-situ carbon coating or encapsulation in mesoporous structures (e.g., FeP/Fe₃O₄ core-shell) is effective. XPS analysis post-synthesis should quantify P(III) (phosphite) and P(V) (phosphate) species, with higher P(0) (phosphide) signals indicating reduced oxidation .
Q. Basic: What characterization techniques are essential for analyzing FeP’s structural and electronic properties?
Methodological Answer:
- XRD: Confirms crystallographic phase (e.g., FeP vs. Fe₂P) using reference patterns (ICDD 00-026-0683).
- TEM/HRTEM: Resolves nanoparticle morphology and lattice fringes (e.g., FeP’s orthorhombic structure).
- XPS: Identifies surface oxidation states (Fe²⁺/Fe³⁺, P⁰/P³⁻) and quantifies oxidized phosphorus species.
- Electrochemical Impedance Spectroscopy (EIS): Measures charge-transfer resistance in HER applications .
Q. Advanced: How do crystallographic facets influence FeP’s hydrogen evolution reaction (HER) activity?
Methodological Answer:
FeP’s HER activity is facet-dependent due to variations in surface energy and active-site density. For example, the (011) facet exhibits lower hydrogen adsorption free energy (ΔG_H*) than (001), as shown by DFT calculations . Experimental validation involves synthesizing single-crystal FeP and comparing Tafel slopes (e.g., 39 mV/dec for nanowires vs. 75 mV/dec for bulk) . In-situ Raman spectroscopy can track H* intermediate adsorption during HER .
Table 2: HER Performance of FeP Nanostructures
Structure | Electrolyte | Tafel Slope (mV/dec) | Overpotential @10 mA/cm² | Reference |
---|---|---|---|---|
FeP Nanowires | 0.5 M H₂SO₄ | 39 | 85 mV | |
FeP/Fe₃O₄ Core-Shell | 1 M KOH | 67 | 120 mV |
Q. Basic: How does FeP’s stability under electrochemical conditions impact long-term HER performance?
Methodological Answer:
FeP undergoes surface oxidation in acidic/alkaline media, forming FeOOH or FePO₄, which increases charge-transfer resistance. Accelerated degradation tests (e.g., 1,000 CV cycles) coupled with post-mortem XPS/TEM reveal oxidation mechanisms. Carbon encapsulation (e.g., ultrathin carbon layers) or doping (e.g., Ni) enhances stability by shielding reactive surfaces .
Q. Advanced: How can phase coexistence (e.g., FeP/Fe₂P) be leveraged to enhance catalytic or battery performance?
Methodological Answer:
Phase boundaries in FeP/Fe₂P heterostructures create synergistic electronic effects. For LIB anodes, FeP’s Li⁺ intercalation (forming LiFeP) and Fe₂P’s conductivity improve rate performance. Operando XRD tracks phase transitions during cycling, while DFT models predict Li⁺ diffusion barriers . In HER, amorphous-crystalline interfaces lower activation energy for H₂O dissociation .
Q. Basic: What safety protocols are critical for handling FeP in laboratory settings?
Methodological Answer:
FeP nanoparticles require inert storage (gloveboxes) to prevent pyrophoric oxidation. PPE includes N95 respirators and flame-resistant lab coats. Spills should be neutralized with sand, not water, to avoid exothermic reactions .
Q. Advanced: How can discrepancies in reported HER activities of FeP be resolved?
Methodological Answer:
Contradictions arise from variations in surface oxidation, electrolyte pH, and testing protocols. Standardization includes:
- Pre-test electrochemical activation (e.g., 50 CV cycles in N₂-saturated electrolyte).
- Reporting overpotential normalized to geometric and electroactive surface area (ECSA).
- Cross-lab validation using reference catalysts (e.g., Pt/C) .
Q. Basic: What are emerging non-HER applications of FeP in materials science?
Methodological Answer:
FeP is explored in:
- Environmental Catalysis: Fenton-like degradation of organic pollutants via •OH generation .
- Battery Anodes: Li⁺/Na⁺ storage with capacities up to 1,500 mAh/g .
- Magnetic Devices: FeP’s metallic conductivity enables spintronic applications .
Q. Advanced: How can atomic pair distribution function (PDF) analysis advance understanding of amorphous FeP phases?
Methodological Answer:
PDF analysis resolves short-range order in amorphous FeP, revealing Fe-P bond distances (~2.3 Å) and coordination numbers. Coupled with reverse Monte Carlo modeling, it identifies precursor phases during crystallization, guiding synthesis of metastable states for enhanced catalysis .
Properties
IUPAC Name |
phosphanylidyneiron | |
---|---|---|
Source | PubChem | |
URL | https://pubchem.ncbi.nlm.nih.gov | |
Description | Data deposited in or computed by PubChem | |
InChI |
InChI=1S/Fe.P | |
Source | PubChem | |
URL | https://pubchem.ncbi.nlm.nih.gov | |
Description | Data deposited in or computed by PubChem | |
InChI Key |
DPTATFGPDCLUTF-UHFFFAOYSA-N | |
Source | PubChem | |
URL | https://pubchem.ncbi.nlm.nih.gov | |
Description | Data deposited in or computed by PubChem | |
Canonical SMILES |
P#[Fe] | |
Source | PubChem | |
URL | https://pubchem.ncbi.nlm.nih.gov | |
Description | Data deposited in or computed by PubChem | |
Molecular Formula |
FeP | |
Record name | iron(III) phosphide | |
Source | Wikipedia | |
URL | https://en.wikipedia.org/wiki/Dictionary_of_chemical_formulas | |
Description | Chemical information link to Wikipedia. | |
Source | PubChem | |
URL | https://pubchem.ncbi.nlm.nih.gov | |
Description | Data deposited in or computed by PubChem | |
DSSTOX Substance ID |
DTXSID5067215 | |
Record name | Iron phosphide (FeP) | |
Source | EPA DSSTox | |
URL | https://comptox.epa.gov/dashboard/DTXSID5067215 | |
Description | DSSTox provides a high quality public chemistry resource for supporting improved predictive toxicology. | |
Molecular Weight |
86.82 g/mol | |
Source | PubChem | |
URL | https://pubchem.ncbi.nlm.nih.gov | |
Description | Data deposited in or computed by PubChem | |
CAS No. |
26508-33-8, 8049-19-2 | |
Record name | Iron phosphide (FeP) | |
Source | CAS Common Chemistry | |
URL | https://commonchemistry.cas.org/detail?cas_rn=26508-33-8 | |
Description | CAS Common Chemistry is an open community resource for accessing chemical information. Nearly 500,000 chemical substances from CAS REGISTRY cover areas of community interest, including common and frequently regulated chemicals, and those relevant to high school and undergraduate chemistry classes. This chemical information, curated by our expert scientists, is provided in alignment with our mission as a division of the American Chemical Society. | |
Explanation | The data from CAS Common Chemistry is provided under a CC-BY-NC 4.0 license, unless otherwise stated. | |
Record name | Iron alloy, base, Fe,P (Ferrophosphorus) | |
Source | CAS Common Chemistry | |
URL | https://commonchemistry.cas.org/detail?cas_rn=8049-19-2 | |
Description | CAS Common Chemistry is an open community resource for accessing chemical information. Nearly 500,000 chemical substances from CAS REGISTRY cover areas of community interest, including common and frequently regulated chemicals, and those relevant to high school and undergraduate chemistry classes. This chemical information, curated by our expert scientists, is provided in alignment with our mission as a division of the American Chemical Society. | |
Explanation | The data from CAS Common Chemistry is provided under a CC-BY-NC 4.0 license, unless otherwise stated. | |
Record name | Iron phosphide (FeP) | |
Source | ChemIDplus | |
URL | https://pubchem.ncbi.nlm.nih.gov/substance/?source=chemidplus&sourceid=0026508338 | |
Description | ChemIDplus is a free, web search system that provides access to the structure and nomenclature authority files used for the identification of chemical substances cited in National Library of Medicine (NLM) databases, including the TOXNET system. | |
Record name | Iron phosphide (FeP) | |
Source | EPA Chemicals under the TSCA | |
URL | https://www.epa.gov/chemicals-under-tsca | |
Description | EPA Chemicals under the Toxic Substances Control Act (TSCA) collection contains information on chemicals and their regulations under TSCA, including non-confidential content from the TSCA Chemical Substance Inventory and Chemical Data Reporting. | |
Record name | Iron phosphide (FeP) | |
Source | EPA DSSTox | |
URL | https://comptox.epa.gov/dashboard/DTXSID5067215 | |
Description | DSSTox provides a high quality public chemistry resource for supporting improved predictive toxicology. | |
Record name | Iron phosphide | |
Source | European Chemicals Agency (ECHA) | |
URL | https://echa.europa.eu/substance-information/-/substanceinfo/100.043.395 | |
Description | The European Chemicals Agency (ECHA) is an agency of the European Union which is the driving force among regulatory authorities in implementing the EU's groundbreaking chemicals legislation for the benefit of human health and the environment as well as for innovation and competitiveness. | |
Explanation | Use of the information, documents and data from the ECHA website is subject to the terms and conditions of this Legal Notice, and subject to other binding limitations provided for under applicable law, the information, documents and data made available on the ECHA website may be reproduced, distributed and/or used, totally or in part, for non-commercial purposes provided that ECHA is acknowledged as the source: "Source: European Chemicals Agency, http://echa.europa.eu/". Such acknowledgement must be included in each copy of the material. ECHA permits and encourages organisations and individuals to create links to the ECHA website under the following cumulative conditions: Links can only be made to webpages that provide a link to the Legal Notice page. | |
Record name | iron phosphide | |
Source | European Chemicals Agency (ECHA) | |
URL | https://echa.europa.eu/information-on-chemicals | |
Description | The European Chemicals Agency (ECHA) is an agency of the European Union which is the driving force among regulatory authorities in implementing the EU's groundbreaking chemicals legislation for the benefit of human health and the environment as well as for innovation and competitiveness. | |
Explanation | Use of the information, documents and data from the ECHA website is subject to the terms and conditions of this Legal Notice, and subject to other binding limitations provided for under applicable law, the information, documents and data made available on the ECHA website may be reproduced, distributed and/or used, totally or in part, for non-commercial purposes provided that ECHA is acknowledged as the source: "Source: European Chemicals Agency, http://echa.europa.eu/". Such acknowledgement must be included in each copy of the material. ECHA permits and encourages organisations and individuals to create links to the ECHA website under the following cumulative conditions: Links can only be made to webpages that provide a link to the Legal Notice page. | |
Disclaimer and Information on In-Vitro Research Products
Please be aware that all articles and product information presented on BenchChem are intended solely for informational purposes. The products available for purchase on BenchChem are specifically designed for in-vitro studies, which are conducted outside of living organisms. In-vitro studies, derived from the Latin term "in glass," involve experiments performed in controlled laboratory settings using cells or tissues. It is important to note that these products are not categorized as medicines or drugs, and they have not received approval from the FDA for the prevention, treatment, or cure of any medical condition, ailment, or disease. We must emphasize that any form of bodily introduction of these products into humans or animals is strictly prohibited by law. It is essential to adhere to these guidelines to ensure compliance with legal and ethical standards in research and experimentation.