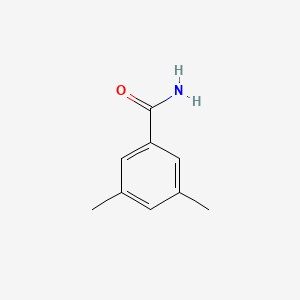
3,5-Dimethylbenzamide
Overview
Description
3,5-Dimethylbenzamide is a benzamide derivative characterized by methyl substituents at the 3- and 5-positions of the benzene ring. This scaffold is widely utilized in medicinal chemistry due to its versatility in forming hydrogen bonds and hydrophobic interactions, making it a key structural motif in enzyme inhibitors and anticancer agents. Derivatives of this compound have demonstrated activity against targets such as histone deacetylases (HDACs) and carbonic anhydrases (CAs), with modifications to the core structure significantly influencing potency, selectivity, and pharmacokinetic properties .
Preparation Methods
Preparation Methods and Analysis
Preparation via Oxidation of Mesitylene to 3,5-Dimethylbenzaldehyde
A key intermediate in the synthesis of 3,5-dimethylbenzamide is 3,5-dimethylbenzaldehyde, which can be prepared by oxidation of mesitylene (1,3,5-trimethylbenzene) under catalytic conditions. The method described in patent CN103524313A involves:
- Mixing divalent cobalt salt and quaternary ammonium bromide salt in mesitylene solvent
- Heating and stirring at 80–110°C to dissolve catalysts
- Introducing oxygen gas at controlled flow rates (0.5 to 1 L/min) at atmospheric or slightly elevated pressure (up to 0.2 MPa)
- Reaction times ranging from 8 to 24 hours at temperatures between 60°C and 160°C
- Post-reaction workup involving pH adjustment (>10) with sodium hydroxide, phase separation, washing, drying, and vacuum distillation to isolate 3,5-dimethylbenzaldehyde at 115–125°C under 20 mmHg pressure
This process achieves selective oxidation of mesitylene methyl groups to the aldehyde functionality with good yield and purity, providing a reliable precursor for amide synthesis.
Step | Conditions | Notes |
---|---|---|
Catalyst mix | Co(II) salt : quaternary ammonium bromide = 1 : 0.5–10 | Stir at room temperature |
Solvent | Mesitylene, 1.4–28 L per mol Co salt | Stir at 400 rpm, heat to 80–110°C |
Oxidation | Oxygen flow: 0.5–1 L/min, 60–160°C, 8–24 h | Atmospheric or 0.2 MPa pressure possible |
Workup | pH >10 with NaOH (1 mol/L), phase separation | Vacuum distillation at 115–125°C/20 mmHg |
Direct Synthesis of this compound via One-Pot Aminolysis
A more direct approach to N-substituted dimethylbenzamides involves the one-pot synthesis starting from 2-amino-3-methylbenzoic acid derivatives. According to research published in the Chinese Journal of Organic Chemistry (2012), the process includes:
- Formation of 8-methyl-2H-3,1-benzoxazine-2,4(1H)-dione intermediate by reacting 2-amino-3-methylbenzoic acid with bis(trichloromethyl) carbonate
- Aminolysis of this intermediate with aqueous methylamine to yield 2-amino-N,3-dimethylbenzamide
- Electrophilic aromatic substitution halogenation (optional) using N-halosuccinimides for functionalized derivatives
This method offers advantages such as:
- No need for intermediate isolation
- High overall yields (87–94%)
- Mild reaction conditions
- Shorter reaction time
- Environmentally friendly (green chemistry approach)
Though this method is reported for halogenated derivatives, the core amide formation step is applicable for preparing this compound analogs.
Comparative Summary of Preparation Methods
Research Findings and Notes
- The oxidation method for 3,5-dimethylbenzaldehyde is well-documented with reproducible conditions involving cobalt catalysts and oxygen under mild pressure, which can be adapted for subsequent amide synthesis.
- The one-pot synthesis approach significantly improves efficiency by eliminating intermediate purification steps, reducing solvent use, and increasing overall yield, aligning with green chemistry principles.
- Pressure-assisted oxidative methods allow for functional group transformations on the aromatic ring, broadening the scope for substituted amides, including this compound derivatives.
- No direct preparation of pure this compound from 3,5-dimethylbenzaldehyde was explicitly found in the sources; however, standard amide synthesis from aldehyde oxidation products or carboxylic acid derivatives via aminolysis or amidation is a common practice in organic synthesis.
Chemical Reactions Analysis
Acylation and Amide Formation
3,5-Dimethylbenzamide is synthesized via amide bond formation between 3,5-dimethylbenzoyl chloride and amines. This reaction is typically conducted in dichloromethane (DCM) or tetrahydrofuran (THF) with a base (e.g., triethylamine) to neutralize HCl byproducts .
Example Reaction:
Key Conditions:
Halogenation Reactions
The ortho-C−H halogenation of this compound derivatives has been achieved using ruthenium catalysts. For example, N,N-diisopropyl-3,5-dimethylbenzamide undergoes bromination with N-bromosuccinimide (NBS) under catalytic Ru₃(CO)₁₂ in 1,2-dichloroethane (DCE) at 120°C .
Example Reaction:
Key Data:
Catalyst | Additive | Conversion (%) | Isolated Yield (%) |
---|---|---|---|
Ru₃(CO)₁₂ | AgO₂CAd | 67 | 62 |
Ru₃(CO)₁₂ | Cu(OAc)₂ | 58 | 58 |
Table 1: Halogenation efficiency under varying conditions .
Cross-Coupling Reactions
Palladium-catalyzed Suzuki-Miyaura cross-coupling enables functionalization of this compound derivatives. For instance, boronate esters of benzamides react with aryl halides to form biaryl structures .
Example Reaction:
Conditions:
Hydrolysis and Functional Group Interconversion
The amide bond in this compound undergoes acid- or base-catalyzed hydrolysis to yield 3,5-dimethylbenzoic acid. This reaction is critical for modifying the compound’s solubility or further derivatization .
Example Reaction:
Key Data:
Deuterium Exchange
Deuterium labeling at the benzamide aromatic ring has been achieved using [IrH₂(Me₂CO)₂(PPh₃)₂]BF₄ as a catalyst under deuterium gas. This method selectively deuterates ortho and para positions relative to the amide group .
Conditions:
Grignard and Organometallic Reactions
This compound reacts with Grignard reagents (e.g., MeMgBr) to form tertiary alcohols or ketones, depending on reaction conditions. For example, methyl substitution on chlorinated derivatives enhances steric and electronic properties .
Example Reaction:
Key Data:
Scientific Research Applications
3,5-Dimethylbenzamide has several scientific research applications:
Chemistry: It is used as an intermediate in the synthesis of various organic compounds.
Biology: It serves as a building block for the development of biologically active molecules.
Medicine: Research is ongoing to explore its potential as a pharmaceutical agent.
Industry: It is used in the production of dyes, pigments, and other industrial chemicals.
Mechanism of Action
The mechanism of action of 3,5-Dimethylbenzamide involves its interaction with specific molecular targets. It can act as an inhibitor or activator of certain enzymes, affecting various biochemical pathways. The exact molecular targets and pathways depend on the specific application and context of its use.
Comparison with Similar Compounds
Selectivity and Mechanism of Action
- LMK235 : The alkoxyamide linker enhances HDAC4/5 selectivity by optimizing interactions with the enzyme’s catalytic pocket, contrasting with pan-HDAC inhibitors like trichostatin A (TSA), which lack linker specificity .
- DM-PIT-1: The carbamothioyl group facilitates interactions with pleckstrin-homology domains, disrupting phosphatidylinositol signaling pathways .
- CA Inhibitors (Compound 24, 26) : Thiourea derivatives exhibit variable inhibition depending on substituent electronegativity; chloro groups (Compound 26) enhance CA IX/XII binding .
Physicochemical and Pharmacokinetic Properties
Research Implications
- HDAC Inhibitors : LMK235’s selectivity profile supports targeted epigenetic therapy, reducing side effects in cancer treatment .
- Carbonic Anhydrase Inhibitors : Substituent optimization (e.g., chloro vs. hydroxyl groups) can enhance isoform-specific activity .
- Anticancer Agents : DM-PIT-1 highlights the importance of structural hybrids (e.g., carbamothioyl-nitro) in disrupting signaling pathways .
Biological Activity
3,5-Dimethylbenzamide is an aromatic amide that has garnered attention in various biological and pharmacological studies. This article explores its biological activity, including its effects on glucose metabolism, potential as an inhibitor for specific enzymes, and its synthesis and applications in medicinal chemistry.
Chemical Structure and Properties
This compound is characterized by the following chemical structure:
- Molecular Formula: C10H13NO
- Molecular Weight: 163.22 g/mol
The compound features a benzene ring with two methyl groups at the 3 and 5 positions and an amide functional group. This configuration contributes to its biological properties and interactions with various biological targets.
Glycogen Phosphorylase Inhibition
One of the notable biological activities of this compound derivatives is their role as glycogen phosphorylase inhibitors. Research has shown that compounds like N-(3,5-Dimethyl-Benzoyl)-N'-(β-D-Glucopyranosyl)urea significantly improve glucose tolerance in both normoglycemic and hyperglycemic conditions. This effect is attributed to the inhibition of glycogen phosphorylase, which plays a critical role in glucose metabolism .
Table 1: Effects of Glycogen Phosphorylase Inhibitors
Compound Name | Effect on Glucose Tolerance | Mechanism of Action |
---|---|---|
N-(3,5-Dimethyl-Benzoyl)-N'-(β-D-Glucopyranosyl)urea | Improved glucose tolerance | Glycogen phosphorylase inhibition |
CP-316819 (Ingliforib) | Reduced glucagon-induced hyperglycemia | Glycogen phosphorylase inhibition |
Inhibition of Carbonic Anhydrases
Another area of research involves the synthesis of sulfonamide-substituted amides that include this compound derivatives. These compounds have been evaluated for their inhibitory effects on human carbonic anhydrases (hCA-II, hCA-IX, hCA-XII). Some derivatives exhibited significant inhibition with IC50 values indicating their potential as therapeutic agents in conditions where carbonic anhydrase activity is implicated .
Table 2: Inhibition Potency of Amide Derivatives
Compound | IC50 Value (µM) | Target Enzyme |
---|---|---|
Compound 9 | 0.18 ± 0.05 | hCA-II |
Compound 11 | 0.17 ± 0.05 | hCA-IX |
Compound 12 | 0.58 ± 0.05 | hCA-XII |
Study on Glucose Handling Disorders
In a study published in PLOS ONE, researchers investigated the metabolic effects of a glucose-derived glycogen phosphorylase inhibitor that included a derivative of this compound. The study demonstrated that this compound not only inhibited glycogen phosphorylase but also enhanced mitochondrial oxidation and mTORC2 signaling pathways to manage glucose influx effectively .
Synthesis and Structure-Activity Relationship (SAR)
The synthesis of various derivatives of this compound has been reported to enhance its biological activity. For example, modifications to the benzoyl moiety can significantly influence the inhibitory potency against target enzymes like carbonic anhydrases. The structure-activity relationship studies highlight how small changes in molecular structure can lead to substantial differences in biological efficacy .
Q & A
Basic Research Questions
Q. How can I optimize the synthesis of 3,5-dimethylbenzamide derivatives for improved yields?
- Methodological Answer : Use multicomponent reactions (MCRs) with tailored reagents. For example, coupling 3,5-dimethylbenzoic acid with isocyanides and formaldehyde via Ugi-type reactions can yield derivatives like N-(2-(butylamino)-2-oxoethyl)-N-(4-(hydroxycarbamoyl)benzyl)-3,5-dimethylbenzamide (68% yield) . Key factors include:
- Temperature control : Maintain 60–80°C during condensation.
- Solvent selection : DMSO or DMF enhances solubility of intermediates.
- Purification : Recrystallization from ethanol/water mixtures improves purity (>98%) .
Q. What spectroscopic techniques are critical for confirming the structure of this compound analogs?
- Methodological Answer : Combine 1H/13C NMR and HRMS for unambiguous characterization. For example:
- 1H NMR (DMSO-d6): Look for aromatic protons (δ 6.96–7.48 ppm) and methyl groups (δ 2.24–2.27 ppm) .
- 13C NMR : Confirm carbonyl signals (δ 163.95–171.6 ppm) and aromatic carbons .
- HRMS : Match experimental and theoretical masses (e.g., 412.2231 [M+H]+ for C23H30N3O4) .
Advanced Research Questions
Q. How do structural modifications to this compound influence histone deacetylase (HDAC) inhibitory activity?
- Methodological Answer : Use structure-activity relationship (SAR) studies to evaluate substituent effects:
- Hydroxamic acid incorporation : Derivatives with N-hydroxycarbamoyl groups (e.g., compound 2j ) show enhanced HDAC1 inhibition (IC50 < 100 nM) due to zinc chelation .
- Alkyl chain length : Longer chains (e.g., butyl in 1x ) improve cellular permeability but may reduce solubility .
- In vitro assays : Measure IC50 values using fluorogenic substrates (e.g., acetylated lysine) and validate with Western blotting for histone H3 acetylation .
Q. What computational methods can predict the stability and reactivity of this compound intermediates?
- Methodological Answer : Apply density functional theory (DFT) with B3LYP/6-311++G(d,p) basis sets to:
- Calculate lattice energies for crystal packing analysis (e.g., hydrogen-bonding networks in acyl hydrazides) .
- Simulate vibrational frequencies (FTIR/Raman) to validate experimental spectra and identify reactive sites .
- Predict hyperpolarizability (β) for nonlinear optical applications .
Q. How can I resolve discrepancies in NMR data for this compound derivatives?
- Methodological Answer : Address contradictions by:
- Solvent standardization : Use deuterated DMSO for consistency in chemical shift reporting .
- Dynamic effects : Account for rotameric splitting (e.g., δ 3.79/3.99 ppm for methylene protons) with variable-temperature NMR .
- Cross-validation : Compare with X-ray crystallography (CCDC 2032776) for absolute configuration confirmation .
Properties
IUPAC Name |
3,5-dimethylbenzamide | |
---|---|---|
Source | PubChem | |
URL | https://pubchem.ncbi.nlm.nih.gov | |
Description | Data deposited in or computed by PubChem | |
InChI |
InChI=1S/C9H11NO/c1-6-3-7(2)5-8(4-6)9(10)11/h3-5H,1-2H3,(H2,10,11) | |
Source | PubChem | |
URL | https://pubchem.ncbi.nlm.nih.gov | |
Description | Data deposited in or computed by PubChem | |
InChI Key |
ZGPFNDFPSMUWJJ-UHFFFAOYSA-N | |
Source | PubChem | |
URL | https://pubchem.ncbi.nlm.nih.gov | |
Description | Data deposited in or computed by PubChem | |
Canonical SMILES |
CC1=CC(=CC(=C1)C(=O)N)C | |
Source | PubChem | |
URL | https://pubchem.ncbi.nlm.nih.gov | |
Description | Data deposited in or computed by PubChem | |
Molecular Formula |
C9H11NO | |
Source | PubChem | |
URL | https://pubchem.ncbi.nlm.nih.gov | |
Description | Data deposited in or computed by PubChem | |
DSSTOX Substance ID |
DTXSID20312874 | |
Record name | 3,5-dimethylbenzamide | |
Source | EPA DSSTox | |
URL | https://comptox.epa.gov/dashboard/DTXSID20312874 | |
Description | DSSTox provides a high quality public chemistry resource for supporting improved predictive toxicology. | |
Molecular Weight |
149.19 g/mol | |
Source | PubChem | |
URL | https://pubchem.ncbi.nlm.nih.gov | |
Description | Data deposited in or computed by PubChem | |
CAS No. |
5692-35-3 | |
Record name | 3,5-Dimethylbenzamide | |
Source | CAS Common Chemistry | |
URL | https://commonchemistry.cas.org/detail?cas_rn=5692-35-3 | |
Description | CAS Common Chemistry is an open community resource for accessing chemical information. Nearly 500,000 chemical substances from CAS REGISTRY cover areas of community interest, including common and frequently regulated chemicals, and those relevant to high school and undergraduate chemistry classes. This chemical information, curated by our expert scientists, is provided in alignment with our mission as a division of the American Chemical Society. | |
Explanation | The data from CAS Common Chemistry is provided under a CC-BY-NC 4.0 license, unless otherwise stated. | |
Record name | 3,5-Dimethylbenzamide | |
Source | ChemIDplus | |
URL | https://pubchem.ncbi.nlm.nih.gov/substance/?source=chemidplus&sourceid=0005692353 | |
Description | ChemIDplus is a free, web search system that provides access to the structure and nomenclature authority files used for the identification of chemical substances cited in National Library of Medicine (NLM) databases, including the TOXNET system. | |
Record name | NSC263781 | |
Source | DTP/NCI | |
URL | https://dtp.cancer.gov/dtpstandard/servlet/dwindex?searchtype=NSC&outputformat=html&searchlist=263781 | |
Description | The NCI Development Therapeutics Program (DTP) provides services and resources to the academic and private-sector research communities worldwide to facilitate the discovery and development of new cancer therapeutic agents. | |
Explanation | Unless otherwise indicated, all text within NCI products is free of copyright and may be reused without our permission. Credit the National Cancer Institute as the source. | |
Record name | 3,5-dimethylbenzamide | |
Source | EPA DSSTox | |
URL | https://comptox.epa.gov/dashboard/DTXSID20312874 | |
Description | DSSTox provides a high quality public chemistry resource for supporting improved predictive toxicology. | |
Synthesis routes and methods
Procedure details
Retrosynthesis Analysis
AI-Powered Synthesis Planning: Our tool employs the Template_relevance Pistachio, Template_relevance Bkms_metabolic, Template_relevance Pistachio_ringbreaker, Template_relevance Reaxys, Template_relevance Reaxys_biocatalysis model, leveraging a vast database of chemical reactions to predict feasible synthetic routes.
One-Step Synthesis Focus: Specifically designed for one-step synthesis, it provides concise and direct routes for your target compounds, streamlining the synthesis process.
Accurate Predictions: Utilizing the extensive PISTACHIO, BKMS_METABOLIC, PISTACHIO_RINGBREAKER, REAXYS, REAXYS_BIOCATALYSIS database, our tool offers high-accuracy predictions, reflecting the latest in chemical research and data.
Strategy Settings
Precursor scoring | Relevance Heuristic |
---|---|
Min. plausibility | 0.01 |
Model | Template_relevance |
Template Set | Pistachio/Bkms_metabolic/Pistachio_ringbreaker/Reaxys/Reaxys_biocatalysis |
Top-N result to add to graph | 6 |
Feasible Synthetic Routes
Disclaimer and Information on In-Vitro Research Products
Please be aware that all articles and product information presented on BenchChem are intended solely for informational purposes. The products available for purchase on BenchChem are specifically designed for in-vitro studies, which are conducted outside of living organisms. In-vitro studies, derived from the Latin term "in glass," involve experiments performed in controlled laboratory settings using cells or tissues. It is important to note that these products are not categorized as medicines or drugs, and they have not received approval from the FDA for the prevention, treatment, or cure of any medical condition, ailment, or disease. We must emphasize that any form of bodily introduction of these products into humans or animals is strictly prohibited by law. It is essential to adhere to these guidelines to ensure compliance with legal and ethical standards in research and experimentation.