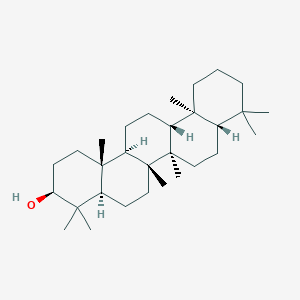
Tetrahymanol
Overview
Description
Tetrahymanol is a polycyclic triterpenoid lipid first discovered in the marine ciliate Tetrahymena pyriformis . It is a gammacerane-type membrane lipid, which has also been found in other ciliates, fungi, ferns, and bacteria . This compound is known for its role in maintaining membrane integrity and is often used as a biomarker for water column stratification in ancient ecosystems .
Preparation Methods
Synthetic Routes and Reaction Conditions: Tetrahymanol is synthesized through the cyclization of squalene. In eukaryotes, this process is catalyzed by the enzyme squalene-tetrahymanol cyclase, which converts squalene directly to this compound . In bacteria, the synthesis involves a distinct pathway where squalene is first converted to a hopene molecule by squalene-hopene cyclase, followed by a Ths-dependent ring expansion to form this compound .
Industrial Production Methods: Industrial production of this compound is not well-documented, but it can be inferred that the process would involve the fermentation of microorganisms capable of producing this compound, followed by extraction and purification of the compound. Genetic engineering techniques could be employed to enhance the yield of this compound in these microorganisms.
Chemical Reactions Analysis
Types of Reactions: Tetrahymanol undergoes various chemical reactions, including oxidation, reduction, and substitution reactions.
Common Reagents and Conditions:
Oxidation: this compound can be oxidized using reagents such as potassium permanganate or chromium trioxide under acidic conditions.
Reduction: Reduction of this compound can be achieved using hydrogen gas in the presence of a palladium catalyst.
Substitution: Substitution reactions can occur at the hydroxyl group of this compound, where it can be replaced by other functional groups using appropriate reagents.
Major Products: The major products formed from these reactions depend on the specific conditions and reagents used. For example, oxidation of this compound can lead to the formation of ketones or carboxylic acids, while reduction can yield fully saturated hydrocarbons.
Scientific Research Applications
Tetrahymanol has a wide range of scientific research applications:
Chemistry: It is used as a model compound to study the biosynthesis of triterpenoids and the mechanisms of cyclization reactions.
Medicine: Research is ongoing to explore the potential therapeutic applications of this compound and its derivatives, particularly in the treatment of diseases related to membrane dysfunction.
Mechanism of Action
Tetrahymanol exerts its effects primarily by integrating into cellular membranes, where it helps to maintain membrane fluidity and stability. In sterol-auxotrophic organisms like Tetrahymena pyriformis, this compound functions as a sterol surrogate, allowing these organisms to thrive in environments where sterol biosynthesis is restricted due to a lack of oxygen . The molecular targets and pathways involved in its mechanism of action include the enzymes responsible for its biosynthesis and the membrane proteins that interact with this compound to maintain membrane integrity.
Comparison with Similar Compounds
Hopanoids: These include diploptene and bacteriohopanetetrol, which are also derived from the gammacerane skeleton.
Sterols: Although not structurally identical, sterols like cholesterol perform similar functions in eukaryotic membranes.
Tetrahymanol’s unique structure and function make it a valuable compound for scientific research and industrial applications.
Properties
IUPAC Name |
(3S,4aR,6aR,6aR,6bR,8aS,12aS,14aR,14bR)-4,4,6a,6b,9,9,12a,14b-octamethyl-1,2,3,4a,5,6,6a,7,8,8a,10,11,12,13,14,14a-hexadecahydropicen-3-ol | |
---|---|---|
Source | PubChem | |
URL | https://pubchem.ncbi.nlm.nih.gov | |
Description | Data deposited in or computed by PubChem | |
InChI |
InChI=1S/C30H52O/c1-25(2)15-9-16-27(5)20(25)12-18-29(7)22(27)10-11-23-28(6)17-14-24(31)26(3,4)21(28)13-19-30(23,29)8/h20-24,31H,9-19H2,1-8H3/t20-,21-,22+,23+,24-,27-,28-,29+,30+/m0/s1 | |
Source | PubChem | |
URL | https://pubchem.ncbi.nlm.nih.gov | |
Description | Data deposited in or computed by PubChem | |
InChI Key |
BFNSRKHIVITRJP-VJBYBJRLSA-N | |
Source | PubChem | |
URL | https://pubchem.ncbi.nlm.nih.gov | |
Description | Data deposited in or computed by PubChem | |
Canonical SMILES |
CC1(CCCC2(C1CCC3(C2CCC4C3(CCC5C4(CCC(C5(C)C)O)C)C)C)C)C | |
Source | PubChem | |
URL | https://pubchem.ncbi.nlm.nih.gov | |
Description | Data deposited in or computed by PubChem | |
Isomeric SMILES |
C[C@]12CCCC([C@@H]1CC[C@@]3([C@@H]2CC[C@H]4[C@]3(CC[C@@H]5[C@@]4(CC[C@@H](C5(C)C)O)C)C)C)(C)C | |
Source | PubChem | |
URL | https://pubchem.ncbi.nlm.nih.gov | |
Description | Data deposited in or computed by PubChem | |
Molecular Formula |
C30H52O | |
Source | PubChem | |
URL | https://pubchem.ncbi.nlm.nih.gov | |
Description | Data deposited in or computed by PubChem | |
DSSTOX Substance ID |
DTXSID60943803 | |
Record name | Tetrahymanol | |
Source | EPA DSSTox | |
URL | https://comptox.epa.gov/dashboard/DTXSID60943803 | |
Description | DSSTox provides a high quality public chemistry resource for supporting improved predictive toxicology. | |
Molecular Weight |
428.7 g/mol | |
Source | PubChem | |
URL | https://pubchem.ncbi.nlm.nih.gov | |
Description | Data deposited in or computed by PubChem | |
Physical Description |
Solid | |
Record name | Tetrahymanol | |
Source | Human Metabolome Database (HMDB) | |
URL | http://www.hmdb.ca/metabolites/HMDB0006836 | |
Description | The Human Metabolome Database (HMDB) is a freely available electronic database containing detailed information about small molecule metabolites found in the human body. | |
Explanation | HMDB is offered to the public as a freely available resource. Use and re-distribution of the data, in whole or in part, for commercial purposes requires explicit permission of the authors and explicit acknowledgment of the source material (HMDB) and the original publication (see the HMDB citing page). We ask that users who download significant portions of the database cite the HMDB paper in any resulting publications. | |
CAS No. |
2130-17-8 | |
Record name | Tetrahymanol | |
Source | CAS Common Chemistry | |
URL | https://commonchemistry.cas.org/detail?cas_rn=2130-17-8 | |
Description | CAS Common Chemistry is an open community resource for accessing chemical information. Nearly 500,000 chemical substances from CAS REGISTRY cover areas of community interest, including common and frequently regulated chemicals, and those relevant to high school and undergraduate chemistry classes. This chemical information, curated by our expert scientists, is provided in alignment with our mission as a division of the American Chemical Society. | |
Explanation | The data from CAS Common Chemistry is provided under a CC-BY-NC 4.0 license, unless otherwise stated. | |
Record name | Tetrahymanol | |
Source | ChemIDplus | |
URL | https://pubchem.ncbi.nlm.nih.gov/substance/?source=chemidplus&sourceid=0002130178 | |
Description | ChemIDplus is a free, web search system that provides access to the structure and nomenclature authority files used for the identification of chemical substances cited in National Library of Medicine (NLM) databases, including the TOXNET system. | |
Record name | Tetrahymanol | |
Source | EPA DSSTox | |
URL | https://comptox.epa.gov/dashboard/DTXSID60943803 | |
Description | DSSTox provides a high quality public chemistry resource for supporting improved predictive toxicology. | |
Record name | Tetrahymanol | |
Source | Human Metabolome Database (HMDB) | |
URL | http://www.hmdb.ca/metabolites/HMDB0006836 | |
Description | The Human Metabolome Database (HMDB) is a freely available electronic database containing detailed information about small molecule metabolites found in the human body. | |
Explanation | HMDB is offered to the public as a freely available resource. Use and re-distribution of the data, in whole or in part, for commercial purposes requires explicit permission of the authors and explicit acknowledgment of the source material (HMDB) and the original publication (see the HMDB citing page). We ask that users who download significant portions of the database cite the HMDB paper in any resulting publications. | |
Retrosynthesis Analysis
AI-Powered Synthesis Planning: Our tool employs the Template_relevance Pistachio, Template_relevance Bkms_metabolic, Template_relevance Pistachio_ringbreaker, Template_relevance Reaxys, Template_relevance Reaxys_biocatalysis model, leveraging a vast database of chemical reactions to predict feasible synthetic routes.
One-Step Synthesis Focus: Specifically designed for one-step synthesis, it provides concise and direct routes for your target compounds, streamlining the synthesis process.
Accurate Predictions: Utilizing the extensive PISTACHIO, BKMS_METABOLIC, PISTACHIO_RINGBREAKER, REAXYS, REAXYS_BIOCATALYSIS database, our tool offers high-accuracy predictions, reflecting the latest in chemical research and data.
Strategy Settings
Precursor scoring | Relevance Heuristic |
---|---|
Min. plausibility | 0.01 |
Model | Template_relevance |
Template Set | Pistachio/Bkms_metabolic/Pistachio_ringbreaker/Reaxys/Reaxys_biocatalysis |
Top-N result to add to graph | 6 |
Feasible Synthetic Routes
Disclaimer and Information on In-Vitro Research Products
Please be aware that all articles and product information presented on BenchChem are intended solely for informational purposes. The products available for purchase on BenchChem are specifically designed for in-vitro studies, which are conducted outside of living organisms. In-vitro studies, derived from the Latin term "in glass," involve experiments performed in controlled laboratory settings using cells or tissues. It is important to note that these products are not categorized as medicines or drugs, and they have not received approval from the FDA for the prevention, treatment, or cure of any medical condition, ailment, or disease. We must emphasize that any form of bodily introduction of these products into humans or animals is strictly prohibited by law. It is essential to adhere to these guidelines to ensure compliance with legal and ethical standards in research and experimentation.