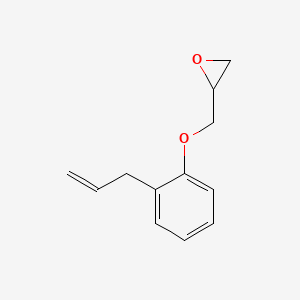
((o-Allylphenoxy)methyl)oxirane
Overview
Description
((o-Allylphenoxy)methyl)oxirane: is an organic compound with the molecular formula C12H14O2 . It is also known by its systematic name, 2-((2-propenyl)phenoxy)methyl)oxirane . This compound is characterized by the presence of an oxirane ring (epoxide) attached to a phenoxy group, which is further substituted with an allyl group. The unique structure of this compound makes it a valuable intermediate in organic synthesis and various industrial applications.
Preparation Methods
Synthetic Routes and Reaction Conditions:
Epoxidation of Allylphenol: One common method for synthesizing ((o-Allylphenoxy)methyl)oxirane involves the epoxidation of allylphenol. This reaction typically uses a peracid, such as m-chloroperoxybenzoic acid (m-CPBA), as the oxidizing agent. The reaction is carried out in an inert solvent like dichloromethane at low temperatures to ensure high selectivity and yield.
Alkylation of Phenol: Another approach involves the alkylation of phenol with allyl bromide to form o-allylphenol, followed by epoxidation using a suitable oxidizing agent.
Industrial Production Methods: In industrial settings, the production of this compound may involve continuous flow reactors to optimize reaction conditions and improve yield. Catalysts such as titanium silicalite-1 (TS-1) can be employed to enhance the efficiency of the epoxidation process.
Chemical Reactions Analysis
Types of Reactions:
Oxidation: ((o-Allylphenoxy)methyl)oxirane can undergo oxidation reactions to form corresponding diols or carbonyl compounds. Common oxidizing agents include hydrogen peroxide and osmium tetroxide.
Reduction: Reduction of the oxirane ring can be achieved using reducing agents like lithium aluminum hydride (LiAlH4) or sodium borohydride (NaBH4), leading to the formation of alcohols.
Substitution: The oxirane ring is highly reactive towards nucleophiles, making it susceptible to ring-opening reactions. Nucleophiles such as amines, thiols, and halides can attack the oxirane ring, resulting in the formation of various substituted products.
Common Reagents and Conditions:
Oxidation: Hydrogen peroxide, osmium tetroxide, dichloromethane, low temperatures.
Reduction: Lithium aluminum hydride, sodium borohydride, tetrahydrofuran (THF), room temperature.
Substitution: Amines, thiols, halides, polar solvents, mild heating.
Major Products Formed:
Oxidation: Diols, carbonyl compounds.
Reduction: Alcohols.
Substitution: Substituted phenoxy compounds.
Scientific Research Applications
Chemistry: ((o-Allylphenoxy)methyl)oxirane is used as a building block in organic synthesis. Its reactivity towards nucleophiles makes it a versatile intermediate for the preparation of complex molecules, including pharmaceuticals and agrochemicals.
Biology and Medicine: In biological research, this compound derivatives are investigated for their potential as enzyme inhibitors and bioactive compounds. Their ability to interact with biological macromolecules makes them valuable tools in drug discovery and development.
Industry: In the industrial sector, this compound is utilized in the production of epoxy resins, which are essential components in coatings, adhesives, and composite materials. Its unique properties contribute to the enhanced performance of these materials.
Mechanism of Action
The mechanism of action of ((o-Allylphenoxy)methyl)oxirane primarily involves its reactivity towards nucleophiles. The strained oxirane ring is prone to nucleophilic attack, leading to ring-opening reactions. This reactivity is exploited in various chemical transformations, where the compound acts as an electrophile. In biological systems, this compound derivatives may interact with enzymes and proteins, potentially inhibiting their activity through covalent modification.
Comparison with Similar Compounds
Phenyl glycidyl ether: Similar to ((o-Allylphenoxy)methyl)oxirane, phenyl glycidyl ether contains an oxirane ring attached to a phenyl group. it lacks the allyl substitution, which affects its reactivity and applications.
Allyl glycidyl ether: This compound features an oxirane ring attached to an allyl group, similar to this compound. it does not contain the phenoxy group, resulting in different chemical properties and uses.
Uniqueness: this compound is unique due to the presence of both allyl and phenoxy groups, which confer distinct reactivity and versatility in chemical synthesis. Its ability to undergo a wide range of reactions makes it a valuable intermediate in various fields, from organic chemistry to industrial applications.
Properties
IUPAC Name |
2-[(2-prop-2-enylphenoxy)methyl]oxirane | |
---|---|---|
Source | PubChem | |
URL | https://pubchem.ncbi.nlm.nih.gov | |
Description | Data deposited in or computed by PubChem | |
InChI |
InChI=1S/C12H14O2/c1-2-5-10-6-3-4-7-12(10)14-9-11-8-13-11/h2-4,6-7,11H,1,5,8-9H2 | |
Source | PubChem | |
URL | https://pubchem.ncbi.nlm.nih.gov | |
Description | Data deposited in or computed by PubChem | |
InChI Key |
XENMLDGAMXHYMH-UHFFFAOYSA-N | |
Source | PubChem | |
URL | https://pubchem.ncbi.nlm.nih.gov | |
Description | Data deposited in or computed by PubChem | |
Canonical SMILES |
C=CCC1=CC=CC=C1OCC2CO2 | |
Source | PubChem | |
URL | https://pubchem.ncbi.nlm.nih.gov | |
Description | Data deposited in or computed by PubChem | |
Molecular Formula |
C12H14O2 | |
Source | PubChem | |
URL | https://pubchem.ncbi.nlm.nih.gov | |
Description | Data deposited in or computed by PubChem | |
Molecular Weight |
190.24 g/mol | |
Source | PubChem | |
URL | https://pubchem.ncbi.nlm.nih.gov | |
Description | Data deposited in or computed by PubChem | |
CAS No. |
4638-04-4 | |
Record name | 2-[[2-(2-Propen-1-yl)phenoxy]methyl]oxirane | |
Source | CAS Common Chemistry | |
URL | https://commonchemistry.cas.org/detail?cas_rn=4638-04-4 | |
Description | CAS Common Chemistry is an open community resource for accessing chemical information. Nearly 500,000 chemical substances from CAS REGISTRY cover areas of community interest, including common and frequently regulated chemicals, and those relevant to high school and undergraduate chemistry classes. This chemical information, curated by our expert scientists, is provided in alignment with our mission as a division of the American Chemical Society. | |
Explanation | The data from CAS Common Chemistry is provided under a CC-BY-NC 4.0 license, unless otherwise stated. | |
Record name | ((o-Allylphenoxy)methyl)oxirane | |
Source | ChemIDplus | |
URL | https://pubchem.ncbi.nlm.nih.gov/substance/?source=chemidplus&sourceid=0004638044 | |
Description | ChemIDplus is a free, web search system that provides access to the structure and nomenclature authority files used for the identification of chemical substances cited in National Library of Medicine (NLM) databases, including the TOXNET system. | |
Record name | NSC130121 | |
Source | DTP/NCI | |
URL | https://dtp.cancer.gov/dtpstandard/servlet/dwindex?searchtype=NSC&outputformat=html&searchlist=130121 | |
Description | The NCI Development Therapeutics Program (DTP) provides services and resources to the academic and private-sector research communities worldwide to facilitate the discovery and development of new cancer therapeutic agents. | |
Explanation | Unless otherwise indicated, all text within NCI products is free of copyright and may be reused without our permission. Credit the National Cancer Institute as the source. | |
Record name | [(o-allylphenoxy)methyl]oxirane | |
Source | European Chemicals Agency (ECHA) | |
URL | https://echa.europa.eu/substance-information/-/substanceinfo/100.022.786 | |
Description | The European Chemicals Agency (ECHA) is an agency of the European Union which is the driving force among regulatory authorities in implementing the EU's groundbreaking chemicals legislation for the benefit of human health and the environment as well as for innovation and competitiveness. | |
Explanation | Use of the information, documents and data from the ECHA website is subject to the terms and conditions of this Legal Notice, and subject to other binding limitations provided for under applicable law, the information, documents and data made available on the ECHA website may be reproduced, distributed and/or used, totally or in part, for non-commercial purposes provided that ECHA is acknowledged as the source: "Source: European Chemicals Agency, http://echa.europa.eu/". Such acknowledgement must be included in each copy of the material. ECHA permits and encourages organisations and individuals to create links to the ECHA website under the following cumulative conditions: Links can only be made to webpages that provide a link to the Legal Notice page. | |
Synthesis routes and methods I
Procedure details
Synthesis routes and methods II
Procedure details
Retrosynthesis Analysis
AI-Powered Synthesis Planning: Our tool employs the Template_relevance Pistachio, Template_relevance Bkms_metabolic, Template_relevance Pistachio_ringbreaker, Template_relevance Reaxys, Template_relevance Reaxys_biocatalysis model, leveraging a vast database of chemical reactions to predict feasible synthetic routes.
One-Step Synthesis Focus: Specifically designed for one-step synthesis, it provides concise and direct routes for your target compounds, streamlining the synthesis process.
Accurate Predictions: Utilizing the extensive PISTACHIO, BKMS_METABOLIC, PISTACHIO_RINGBREAKER, REAXYS, REAXYS_BIOCATALYSIS database, our tool offers high-accuracy predictions, reflecting the latest in chemical research and data.
Strategy Settings
Precursor scoring | Relevance Heuristic |
---|---|
Min. plausibility | 0.01 |
Model | Template_relevance |
Template Set | Pistachio/Bkms_metabolic/Pistachio_ringbreaker/Reaxys/Reaxys_biocatalysis |
Top-N result to add to graph | 6 |
Feasible Synthetic Routes
Disclaimer and Information on In-Vitro Research Products
Please be aware that all articles and product information presented on BenchChem are intended solely for informational purposes. The products available for purchase on BenchChem are specifically designed for in-vitro studies, which are conducted outside of living organisms. In-vitro studies, derived from the Latin term "in glass," involve experiments performed in controlled laboratory settings using cells or tissues. It is important to note that these products are not categorized as medicines or drugs, and they have not received approval from the FDA for the prevention, treatment, or cure of any medical condition, ailment, or disease. We must emphasize that any form of bodily introduction of these products into humans or animals is strictly prohibited by law. It is essential to adhere to these guidelines to ensure compliance with legal and ethical standards in research and experimentation.