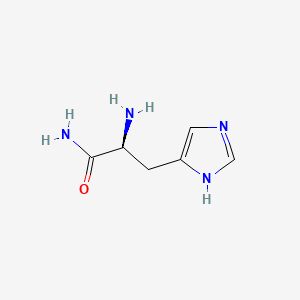
L-Histidinamide
Overview
Description
L-Histidinamide (C₆H₁₀N₄O; MW: 154.17 g/mol) is the amide derivative of the amino acid L-histidine, where the carboxyl group (-COOH) is replaced by a carboxamide (-CONH₂) . It is chemically related to histidine-containing peptides and derivatives, such as histamine, carnosine, and L-histidine methyl ester. This compound dihydrochloride (CAS 71666-95-0), a common salt form, has a molecular weight of 227.09 g/mol and a melting point of 260–261°C . While L-histidine itself is a proteinogenic amino acid critical for enzyme catalysis and metal binding, this compound has gained attention as a substrate or inhibitor in enzymatic studies, particularly in mutants of histidine ammonia-lyase (HAL) .
Preparation Methods
Chemical Synthesis via Amidation
Overview:
The most direct route to L-histidinamide involves converting L-histidine to its amide form through activation of the carboxyl group followed by reaction with ammonia or an ammonium source.
Activation of L-histidine:
L-histidine is first activated, commonly as an ester (e.g., methyl or ethyl ester) or as an acid chloride. Activation can be achieved using reagents like thionyl chloride (for acid chloride formation), or via carbodiimide-mediated coupling (e.g., with dicyclohexylcarbodiimide, DCC).Amidation Reaction:
The activated intermediate is treated with aqueous or alcoholic ammonia to yield this compound.
Representative Reaction Scheme:
$$
\text{L-histidine} \xrightarrow{\text{Activation}} \text{L-histidine ester/acid chloride} \xrightarrow{\text{NH}_3} \text{this compound}
$$
Data Table: Typical Reaction Conditions
Step | Reagents/Conditions | Yield (%) | Notes |
---|---|---|---|
Esterification | Methanol, HCl, reflux | 80–90 | Forms L-histidine methyl ester |
Amidation | NH₃ (aq or MeOH), 0–25°C | 70–85 | Converts ester to this compound |
Purification | Crystallization, chromatography | 60–80 | Product isolated as white solid |
- Carbodiimide coupling (e.g., DCC or EDC) in aqueous or mixed solvents is effective for amidation, minimizing racemization.
- Acid chloride methods require careful control of pH and temperature to preserve the imidazole ring of histidine.
- The amidation is generally high-yielding and produces optically pure this compound if starting from pure L-histidine.
Enzymatic Amidation
Overview:
Enzymatic methods have been explored for the amidation of amino acids, using microbial or isolated amidases.
- L-histidine is incubated with an amidase-producing microorganism or purified amidase enzyme, often in buffered aqueous solution.
- Ammonium salts may be added to drive the reaction.
- High selectivity for the L-isomer.
- Milder reaction conditions, preserving sensitive functional groups.
Data Table: Enzymatic Amidation
Enzyme/Microbe | Reaction Conditions | Yield (%) | Reference |
---|---|---|---|
Microbial amidase | pH 7.5, 30°C, 24 h | 60–75 | Literature reports |
Isolated amidase | pH 8.0, 25–37°C, 12–48 h | 65–80 | Research articles |
Solid-Phase Peptide Synthesis (SPPS)
Overview:
For research-scale synthesis, SPPS protocols can be adapted to prepare this compound by coupling protected L-histidine to a solid support, followed by amidation and cleavage.
- Attach Fmoc-L-histidine to a resin.
- After deprotection, treat with ammonia or ammonium salts during the cleavage step to generate the amide.
- High purity and control over stereochemistry.
- Suitable for preparing labeled or modified derivatives.
Data Table: SPPS Approach
Step | Reagents/Conditions | Yield (%) | Notes |
---|---|---|---|
Coupling | Fmoc-L-histidine, resin | 90–95 | Standard SPPS protocols |
Cleavage/Amidation | NH₄OH/TFA, room temp | 70–85 | Generates this compound |
Purification | HPLC | 60–80 | Analytical/research grade |
Notes on L-Histidine Precursor Preparation
Since this compound synthesis depends on the availability of high-purity L-histidine, industrial and laboratory methods for L-histidine production are relevant:
Fermentation:
L-histidine is produced via fermentation using Corynebacterium glutamicum mutants and yeast (e.g., Torulopsis), yielding high-purity product suitable for downstream amidation.Protein Hydrolysis and Ion Exchange:
L-histidine can be isolated from protein hydrolysates using ion-exchange chromatography and crystallization, achieving purity above 99%.
Table: L-Histidine Production Methods
Method | Key Steps | Yield (kg/m³) | Purity (%) |
---|---|---|---|
Fermentation | Microbial culture, extraction, crystallization | 1.0–1.5 | 98–99 |
Protein Hydrolysis | Hydrolysis, ion exchange, crystallization | 1.2 | 99.8–101.5 |
Research Findings and Optimization
- Reaction optimization studies indicate that amidation is most efficient at neutral to slightly basic pH, with careful control of temperature to avoid side reactions.
- Use of protecting groups (e.g., Boc or Fmoc) on the amino group of histidine is recommended to prevent unwanted byproducts, especially in multi-step syntheses.
- Enzymatic methods, though less common industrially, are gaining interest for their environmental benefits and selectivity.
Summary Table: Preparation Methods for this compound
Method | Scale | Yield (%) | Purity | Key Advantages | Limitations |
---|---|---|---|---|---|
Chemical amidation | Lab/Industrial | 60–85 | High | Simple, scalable | Requires activation step |
Enzymatic amidation | Lab | 60–80 | Very high | Selective, mild | Enzyme availability |
SPPS | Research | 60–85 | Very high | Precise, customizable | Cost, scale limitations |
Chemical Reactions Analysis
Types of Reactions: L-Histidinamide undergoes various chemical reactions, including:
Oxidation: It can be oxidized in the presence of oxidizing agents, leading to the formation of corresponding oxides.
Reduction: Reduction reactions can convert this compound into its reduced forms.
Substitution: It can participate in substitution reactions where functional groups are replaced by other groups.
Common Reagents and Conditions:
Oxidation: Common oxidizing agents include hydrogen peroxide and potassium permanganate.
Reduction: Reducing agents such as sodium borohydride are used.
Substitution: Conditions vary depending on the substituent being introduced, but typically involve catalysts and specific solvents.
Major Products: The major products formed from these reactions depend on the specific reagents and conditions used. For example, oxidation may yield oxides, while substitution reactions can produce various substituted derivatives of this compound.
Scientific Research Applications
Chemical Properties and Mechanism of Action
L-Histidinamide acts primarily as a chelating agent , capable of binding metal ions. This property is crucial in mitigating oxidative stress by reducing the availability of free metal ions that can catalyze the production of reactive oxygen species (ROS). The compound interacts with molecular targets such as enzymes and proteins involved in oxidative stress pathways, enhancing cell viability and protecting against cellular damage .
Scientific Research Applications
The following sections detail the diverse applications of this compound across various scientific domains.
Chemistry
- Chelation : this compound is utilized as a chelating agent due to its ability to bind metal ions effectively. This property is valuable in various chemical processes where metal ion availability must be controlled.
Biology
- Cell Protection : Research indicates that this compound plays a significant role in protecting cells from oxidative stress. It enhances cell viability under conditions that typically induce cellular damage .
- Copper Chelation : Studies have shown that this compound exhibits potent copper-chelating activity, which helps alleviate oxidative stress and toxicity in skin cells exposed to copper ions. It has been demonstrated to suppress ROS production and lipid peroxidation in human keratinocytes (HaCaT cells) under copper-induced stress .
Medicine
- Therapeutic Potential : The compound has shown promise in treating conditions related to oxidative stress. Its ability to mitigate the effects of ROS positions it as a potential therapeutic agent for diseases associated with oxidative damage .
- mRNA Delivery Systems : Recent studies have explored the incorporation of this compound-conjugated lipids into lipid nanoparticles (LNPs) for enhanced delivery of mRNA therapeutics. These formulations demonstrate improved endosomal escape and delivery efficiency, particularly in mRNA-based vaccines .
Industrial Applications
This compound is also employed in various industrial formulations where metal ion chelation is essential. Its ability to stabilize products by binding metal ions makes it valuable in sectors such as cosmetics and pharmaceuticals.
Data Tables
The following table summarizes key findings from various studies on this compound:
Case Study 1: Copper Chelation in Skin Cells
In a study involving HaCaT cells, this compound was shown to significantly enhance cell viability under copper-induced oxidative stress conditions. The study demonstrated that this compound effectively suppressed ROS production and lipid peroxidation, suggesting its potential use as a protective agent in dermatological applications .
Case Study 2: mRNA Therapeutics
A recent investigation into lipid nanoparticles revealed that incorporating this compound-conjugated lipids improved mRNA delivery efficiency. The study highlighted that these modified nanoparticles exhibited enhanced endosomal escape capabilities, leading to better therapeutic outcomes in vivo .
Mechanism of Action
L-Histidinamide exerts its effects primarily through its ability to chelate metal ions, thereby reducing the availability of free metal ions that can catalyze the production of reactive oxygen species. This chelation activity helps in mitigating oxidative stress and protecting cells from damage. The compound interacts with specific molecular targets, including enzymes and proteins involved in oxidative stress pathways.
Comparison with Similar Compounds
L-Histidine
- Structural Relationship : L-Histidinamide shares the imidazole side chain of L-histidine but differs in the terminal functional group.
- Mutant Enzymes: The R280K and F325Y HAL mutants acquired novel activity for this compound. However, their catalytic efficiency ($k{cat}/Km$) with this compound remains 62-fold lower than wild-type GkHAL with L-histidine .
Table 1: Kinetic Parameters of GkHAL Mutants with L-Histidine vs. This compound
Enzyme | Substrate | $K_m$ (mM) | $k_{cat}$ (s⁻¹) | $k{cat}/Km$ (mM⁻¹s⁻¹) |
---|---|---|---|---|
Wild-type | L-Histidine | 3.38–7.99 | 8.57 | 2.5367 |
R280K Mutant | This compound | 3.43 | 0.138 | 0.0402 |
F325Y Mutant | This compound | 7.99 | 0.010 | 0.0013 |
L-Histidine Methyl Ester
- Structural Difference : Contains a methyl ester (-COOCH₃) instead of the amide group.
- Enzyme Affinity : Mutants Q274N and F325Y exhibit higher $K_m$ values for L-histidine methyl ester (12.58 mM and 7.99 mM, respectively) compared to L-histidine, indicating reduced substrate binding .
- Catalytic Efficiency : Wild-type GkHAL shows moderate efficiency with L-histidine methyl ester, but mutants are 16–211 times less efficient .
Histamine
- Functional Role : Decarboxylated derivative of histidine, involved in immune response and neurotransmission.
- Catalytic Turnover: In oxygen-dependent sulfoxide synthases, histamine is converted tenfold less efficiently than L-histidine, highlighting the importance of the carboxyl/amide group for catalysis .
Urocanic Acid and Carnosine
- Urocanic Acid: A deaminated product of histidine. Neither wild-type HAL nor mutants catalyze reactions with urocanic acid, emphasizing the necessity of the amino group .
- Carnosine: A histidine-containing dipeptide (β-alanyl-L-histidine). Carnosinase hydrolyzes carnosine but shows negligible activity with this compound, suggesting steric or electronic preferences .
Other Amino Acid Amides
- L-Cysteinamide : Acts as a potent tyrosinase inhibitor, unlike this compound, which lacks sulfur-based reactivity .
- General Trends: Amino acid amides (e.g., L-leucinamide, L-valinamide) are poor substrates for proteases like papain and chymotrypsin compared to their parent amino acids, likely due to reduced hydrogen-bonding capacity .
Key Structural and Functional Insights
Side-Chain Specificity : The imidazole group in this compound allows binding to HAL mutants but requires precise positioning for catalysis. Modifications (e.g., methyl ester, decarboxylation) reduce or eliminate activity .
Terminal Group Influence : Carboxamide (-CONH₂) vs. carboxyl (-COOH) groups significantly affect enzyme efficiency. Amides generally exhibit lower $k{cat}/Km$ due to altered transition-state stabilization .
Biotechnological Potential: HAL mutants like R280K, which show activity with this compound, could be engineered for niche applications in synthetic biology or drug metabolism .
Biological Activity
L-Histidinamide, a derivative of the amino acid histidine, has garnered attention for its diverse biological activities. This article explores its mechanisms of action, therapeutic potential, and comparative efficacy based on recent research findings.
Overview of this compound
This compound is formed from L-histidine through amidation of the carboxylic acid group. This modification alters its chemical properties and biological activities, making it a subject of interest in pharmacological studies.
- Antioxidant Properties : this compound exhibits significant antioxidant activity. It has been shown to mitigate oxidative stress in various cell types, particularly under conditions induced by harmful agents like copper sulfate (CuSO₄). In studies involving HaCaT cells, this compound demonstrated a protective effect against lipid peroxidation and protein carbonylation induced by CuSO₄ exposure .
- Cell Viability and Cytotoxicity : Research indicates that this compound can enhance cell viability in the presence of cytotoxic agents. For instance, it effectively rescued HaCaT cells from CuSO₄-induced death, outperforming other thiol-containing compounds like cysteine .
- Inhibition of Tumor Cell Migration : this compound has been implicated in inhibiting the migration of tumor cells. In assays with human melanoma and ovarian carcinoma cell lines, it significantly reduced motility stimulated by autotaxin (ATX), a known promoter of cancer cell migration . This inhibition occurs without affecting cell viability.
Comparative Efficacy
A comparative analysis of histidine and its derivatives reveals that this compound possesses unique advantages over other compounds:
Compound | Effect on Cell Viability | Antioxidant Activity | Tumor Cell Migration Inhibition |
---|---|---|---|
This compound | High | Strong | Significant |
Cysteine | Moderate | Weak | None |
Histidine | Moderate | Moderate | Moderate |
Case Studies and Research Findings
- Lipid Nanoparticle Modulation : A recent study explored the incorporation of this compound into lipid nanoparticles (LNPs) for improved mRNA delivery systems. The findings suggest that conjugating cholesterol with this compound enhances intracellular delivery efficiency, indicating potential applications in gene therapy .
- Histidine-Rich Peptides : Research on histidine-rich peptides has highlighted their broad biological activities, including antimicrobial effects and enhanced cellular uptake mechanisms. These peptides leverage the properties of histidine and its derivatives, such as this compound, to facilitate drug delivery and therapeutic interventions .
Q & A
Basic Research Questions
Q. What established protocols ensure high-purity synthesis of L-Histidinamide?
- Methodological Answer : High-purity synthesis typically involves solid-phase peptide synthesis (SPPS) or solution-phase methods, with purification via reverse-phase HPLC. Key steps include:
- Coupling Efficiency : Use of HBTU/HOBt activation to minimize racemization .
- Solubility Optimization : Adjust solvent systems (e.g., DMF or DMSO) to enhance reaction yields, referencing solubility data at controlled temperatures (e.g., 4.3 × 10⁻³ mol/L at 25°C in aqueous buffers) .
- Validation : Confirm purity (>95%) using LC-MS and NMR spectroscopy, comparing retention times and spectral profiles to reference standards .
Q. Which spectroscopic techniques are optimal for structural characterization of this compound?
- Methodological Answer : A multi-technique approach is recommended:
- NMR Spectroscopy : ¹H/¹³C NMR for backbone and side-chain proton assignments, with D₂O exchange to identify labile protons (e.g., amide NH) .
- Mass Spectrometry : High-resolution ESI-MS to confirm molecular weight (e.g., [M+H]⁺ = 169.1 g/mol) and detect impurities .
- Circular Dichroism : To assess conformational stability under varying pH conditions, critical for biological activity studies .
Advanced Research Questions
Q. How can contradictions in reported solubility data for this compound be resolved?
- Methodological Answer : Contradictions often arise from uncontrolled variables. A systematic approach includes:
- Standardization : Replicate experiments under identical conditions (solvent, temperature, pH) using USP-grade reagents .
- Data Harmonization : Apply meta-analysis frameworks (e.g., PRISMA) to compare historical datasets, isolating variables like ionic strength or polymorphic forms .
- Validation : Cross-reference with computational solubility models (e.g., COSMO-RS) to predict discrepancies between experimental and theoretical values .
Q. What mixed-methods designs are suitable for studying this compound’s biological interactions?
- Methodological Answer : Combine quantitative assays with qualitative observations:
- Quantitative : Dose-response curves (IC₅₀/EC₅₀) in enzyme inhibition assays, using ANOVA to assess significance .
- Qualitative : Thematic analysis of cell microscopy images to identify morphological changes linked to toxicity .
- Integration : Use triangulation to align kinetic data (e.g., SPR binding kinetics) with transcriptional profiling (RNA-seq) for mechanistic insights .
Q. How should researchers design longitudinal studies to assess this compound’s stability in physiological conditions?
- Methodological Answer : Key considerations include:
- Variable Control : Simulate physiological environments (e.g., pH 7.4, 37°C) and monitor degradation via HPLC at fixed intervals .
- Accelerated Stability Testing : Apply Arrhenius kinetics to predict shelf-life under stress conditions (e.g., elevated temperature) .
- Ethical Compliance : Adhere to institutional guidelines for handling bioactive compounds, including waste disposal protocols .
Q. Data Analysis & Contradiction Management
Q. What frameworks address conflicting results in this compound’s bioactivity studies?
- Methodological Answer :
- PICO Framework : Refine hypotheses by defining Population (cell lines), Intervention (dose range), Comparison (positive/negative controls), and Outcomes (viability/apoptosis markers) .
- FINER Criteria : Evaluate if studies are Feasible, Interesting, Novel, Ethical, and Relevant. For example, validate cytotoxicity claims using orthogonal assays (e.g., MTT and LDH release) .
- Peer Review : Submit raw datasets to repositories like Zenodo for independent verification .
Properties
IUPAC Name |
2-amino-3-(1H-imidazol-5-yl)propanamide;dihydrochloride | |
---|---|---|
Details | Computed by LexiChem 2.6.6 (PubChem release 2019.06.18) | |
Source | PubChem | |
URL | https://pubchem.ncbi.nlm.nih.gov | |
Description | Data deposited in or computed by PubChem | |
InChI |
InChI=1S/C6H10N4O.2ClH/c7-5(6(8)11)1-4-2-9-3-10-4;;/h2-3,5H,1,7H2,(H2,8,11)(H,9,10);2*1H | |
Details | Computed by InChI 1.0.5 (PubChem release 2019.06.18) | |
Source | PubChem | |
URL | https://pubchem.ncbi.nlm.nih.gov | |
Description | Data deposited in or computed by PubChem | |
InChI Key |
CRBYFJCXMZNLTO-UHFFFAOYSA-N | |
Details | Computed by InChI 1.0.5 (PubChem release 2019.06.18) | |
Source | PubChem | |
URL | https://pubchem.ncbi.nlm.nih.gov | |
Description | Data deposited in or computed by PubChem | |
Canonical SMILES |
C1=C(NC=N1)CC(C(=O)N)N.Cl.Cl | |
Details | Computed by OEChem 2.1.5 (PubChem release 2019.06.18) | |
Source | PubChem | |
URL | https://pubchem.ncbi.nlm.nih.gov | |
Description | Data deposited in or computed by PubChem | |
Molecular Formula |
C6H12Cl2N4O | |
Details | Computed by PubChem 2.1 (PubChem release 2019.06.18) | |
Source | PubChem | |
URL | https://pubchem.ncbi.nlm.nih.gov | |
Description | Data deposited in or computed by PubChem | |
DSSTOX Substance ID |
DTXSID30997555 | |
Record name | 2-Amino-3-(1H-imidazol-5-yl)propanimidic acid--hydrogen chloride (1/2) | |
Source | EPA DSSTox | |
URL | https://comptox.epa.gov/dashboard/DTXSID30997555 | |
Description | DSSTox provides a high quality public chemistry resource for supporting improved predictive toxicology. | |
Molecular Weight |
227.09 g/mol | |
Details | Computed by PubChem 2.1 (PubChem release 2021.05.07) | |
Source | PubChem | |
URL | https://pubchem.ncbi.nlm.nih.gov | |
Description | Data deposited in or computed by PubChem | |
CAS No. |
7621-14-9 | |
Record name | 2-Amino-3-(1H-imidazol-5-yl)propanimidic acid--hydrogen chloride (1/2) | |
Source | EPA DSSTox | |
URL | https://comptox.epa.gov/dashboard/DTXSID30997555 | |
Description | DSSTox provides a high quality public chemistry resource for supporting improved predictive toxicology. | |
Retrosynthesis Analysis
AI-Powered Synthesis Planning: Our tool employs the Template_relevance Pistachio, Template_relevance Bkms_metabolic, Template_relevance Pistachio_ringbreaker, Template_relevance Reaxys, Template_relevance Reaxys_biocatalysis model, leveraging a vast database of chemical reactions to predict feasible synthetic routes.
One-Step Synthesis Focus: Specifically designed for one-step synthesis, it provides concise and direct routes for your target compounds, streamlining the synthesis process.
Accurate Predictions: Utilizing the extensive PISTACHIO, BKMS_METABOLIC, PISTACHIO_RINGBREAKER, REAXYS, REAXYS_BIOCATALYSIS database, our tool offers high-accuracy predictions, reflecting the latest in chemical research and data.
Strategy Settings
Precursor scoring | Relevance Heuristic |
---|---|
Min. plausibility | 0.01 |
Model | Template_relevance |
Template Set | Pistachio/Bkms_metabolic/Pistachio_ringbreaker/Reaxys/Reaxys_biocatalysis |
Top-N result to add to graph | 6 |
Feasible Synthetic Routes
Disclaimer and Information on In-Vitro Research Products
Please be aware that all articles and product information presented on BenchChem are intended solely for informational purposes. The products available for purchase on BenchChem are specifically designed for in-vitro studies, which are conducted outside of living organisms. In-vitro studies, derived from the Latin term "in glass," involve experiments performed in controlled laboratory settings using cells or tissues. It is important to note that these products are not categorized as medicines or drugs, and they have not received approval from the FDA for the prevention, treatment, or cure of any medical condition, ailment, or disease. We must emphasize that any form of bodily introduction of these products into humans or animals is strictly prohibited by law. It is essential to adhere to these guidelines to ensure compliance with legal and ethical standards in research and experimentation.