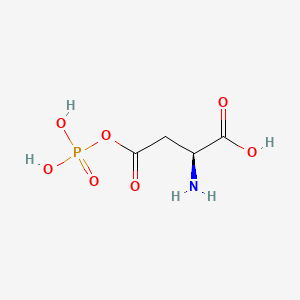
Aspartyl phosphate
Overview
Description
Preparation Methods
Phosphoaspartate can be synthesized through various methods. One common approach involves the phosphorylation of aspartic acid using phosphorylating agents under controlled conditions. The reaction typically requires acidic conditions to facilitate the nucleophilic attack on the aspartate carbonyl carbon atom . Industrial production methods often involve the use of reductive cleavage with sodium borohydride (NaBH4) to detect free or protein-bound phosphoaspartate .
Chemical Reactions Analysis
Phosphoaspartate undergoes several types of chemical reactions, including:
Oxidation: Phosphoaspartate can be oxidized under specific conditions, leading to the formation of various oxidized products.
Reduction: The compound can be reduced using reducing agents such as sodium borohydride.
Substitution: Phosphoaspartate can participate in substitution reactions, where the phosphoryl group is replaced by other functional groups.
Common reagents used in these reactions include phosphorylating agents, reducing agents like sodium borohydride, and various acids and bases to control the reaction conditions . The major products formed from these reactions depend on the specific reagents and conditions used.
Scientific Research Applications
Phosphoaspartate has a wide range of scientific research applications, including:
Chemistry: It is used as a model compound to study phosphorylation and dephosphorylation reactions.
Biology: Phosphoaspartate is involved in two-component signaling pathways in prokaryotes, where it acts as a key intermediate in the transfer of phosphate groups.
Medicine: Research on phosphoaspartate contributes to understanding cellular signaling mechanisms and developing therapeutic strategies targeting phosphorylation pathways.
Industry: The compound is used in the production of various biochemical reagents and as a tool for studying enzyme mechanisms .
Mechanism of Action
Phosphoaspartate exerts its effects through its role in phosphorylation and dephosphorylation reactions. The general mechanism involves the nucleophilic attack of an oxygen nucleophile on the phosphorus atom in the key phosphoaspartate residue. This reaction is crucial for the function of ATPases, phosphatases, β-phosphoglucomutase, and phosphonoacetaldehyde hydrolase enzymes .
Comparison with Similar Compounds
Phosphoaspartate is similar to other phosphoamino acids such as phosphoglutamate (γ-glutamyl phosphate), phosphoserine, phosphothreonine, and phosphotyrosine. it is unique in its role as a major component of prokaryotic two-component signaling pathways and its involvement in specific enzymatic reactions . Similar compounds include:
- Phosphoglutamate
- Phosphoserine
- Phosphothreonine
- Phosphotyrosine
These compounds share similar chemical properties but differ in their specific biological roles and mechanisms of action.
Properties
IUPAC Name |
(2S)-2-amino-4-oxo-4-phosphonooxybutanoic acid | |
---|---|---|
Source | PubChem | |
URL | https://pubchem.ncbi.nlm.nih.gov | |
Description | Data deposited in or computed by PubChem | |
InChI |
InChI=1S/C4H8NO7P/c5-2(4(7)8)1-3(6)12-13(9,10)11/h2H,1,5H2,(H,7,8)(H2,9,10,11)/t2-/m0/s1 | |
Source | PubChem | |
URL | https://pubchem.ncbi.nlm.nih.gov | |
Description | Data deposited in or computed by PubChem | |
InChI Key |
IXZNKTPIYKDIGG-REOHCLBHSA-N | |
Source | PubChem | |
URL | https://pubchem.ncbi.nlm.nih.gov | |
Description | Data deposited in or computed by PubChem | |
Canonical SMILES |
C(C(C(=O)O)N)C(=O)OP(=O)(O)O | |
Source | PubChem | |
URL | https://pubchem.ncbi.nlm.nih.gov | |
Description | Data deposited in or computed by PubChem | |
Isomeric SMILES |
C([C@@H](C(=O)O)N)C(=O)OP(=O)(O)O | |
Source | PubChem | |
URL | https://pubchem.ncbi.nlm.nih.gov | |
Description | Data deposited in or computed by PubChem | |
Molecular Formula |
C4H8NO7P | |
Source | PubChem | |
URL | https://pubchem.ncbi.nlm.nih.gov | |
Description | Data deposited in or computed by PubChem | |
Molecular Weight |
213.08 g/mol | |
Source | PubChem | |
URL | https://pubchem.ncbi.nlm.nih.gov | |
Description | Data deposited in or computed by PubChem | |
Physical Description |
Solid | |
Record name | L-Aspartyl-4-phosphate | |
Source | Human Metabolome Database (HMDB) | |
URL | http://www.hmdb.ca/metabolites/HMDB0012250 | |
Description | The Human Metabolome Database (HMDB) is a freely available electronic database containing detailed information about small molecule metabolites found in the human body. | |
Explanation | HMDB is offered to the public as a freely available resource. Use and re-distribution of the data, in whole or in part, for commercial purposes requires explicit permission of the authors and explicit acknowledgment of the source material (HMDB) and the original publication (see the HMDB citing page). We ask that users who download significant portions of the database cite the HMDB paper in any resulting publications. | |
CAS No. |
22138-53-0 | |
Record name | β-Aspartyl phosphate | |
Source | CAS Common Chemistry | |
URL | https://commonchemistry.cas.org/detail?cas_rn=22138-53-0 | |
Description | CAS Common Chemistry is an open community resource for accessing chemical information. Nearly 500,000 chemical substances from CAS REGISTRY cover areas of community interest, including common and frequently regulated chemicals, and those relevant to high school and undergraduate chemistry classes. This chemical information, curated by our expert scientists, is provided in alignment with our mission as a division of the American Chemical Society. | |
Explanation | The data from CAS Common Chemistry is provided under a CC-BY-NC 4.0 license, unless otherwise stated. | |
Record name | beta-Aspartyl phosphate | |
Source | ChemIDplus | |
URL | https://pubchem.ncbi.nlm.nih.gov/substance/?source=chemidplus&sourceid=0022138530 | |
Description | ChemIDplus is a free, web search system that provides access to the structure and nomenclature authority files used for the identification of chemical substances cited in National Library of Medicine (NLM) databases, including the TOXNET system. | |
Record name | L-Aspartyl-4-phosphate | |
Source | Human Metabolome Database (HMDB) | |
URL | http://www.hmdb.ca/metabolites/HMDB0012250 | |
Description | The Human Metabolome Database (HMDB) is a freely available electronic database containing detailed information about small molecule metabolites found in the human body. | |
Explanation | HMDB is offered to the public as a freely available resource. Use and re-distribution of the data, in whole or in part, for commercial purposes requires explicit permission of the authors and explicit acknowledgment of the source material (HMDB) and the original publication (see the HMDB citing page). We ask that users who download significant portions of the database cite the HMDB paper in any resulting publications. | |
Retrosynthesis Analysis
AI-Powered Synthesis Planning: Our tool employs the Template_relevance Pistachio, Template_relevance Bkms_metabolic, Template_relevance Pistachio_ringbreaker, Template_relevance Reaxys, Template_relevance Reaxys_biocatalysis model, leveraging a vast database of chemical reactions to predict feasible synthetic routes.
One-Step Synthesis Focus: Specifically designed for one-step synthesis, it provides concise and direct routes for your target compounds, streamlining the synthesis process.
Accurate Predictions: Utilizing the extensive PISTACHIO, BKMS_METABOLIC, PISTACHIO_RINGBREAKER, REAXYS, REAXYS_BIOCATALYSIS database, our tool offers high-accuracy predictions, reflecting the latest in chemical research and data.
Strategy Settings
Precursor scoring | Relevance Heuristic |
---|---|
Min. plausibility | 0.01 |
Model | Template_relevance |
Template Set | Pistachio/Bkms_metabolic/Pistachio_ringbreaker/Reaxys/Reaxys_biocatalysis |
Top-N result to add to graph | 6 |
Feasible Synthetic Routes
Disclaimer and Information on In-Vitro Research Products
Please be aware that all articles and product information presented on BenchChem are intended solely for informational purposes. The products available for purchase on BenchChem are specifically designed for in-vitro studies, which are conducted outside of living organisms. In-vitro studies, derived from the Latin term "in glass," involve experiments performed in controlled laboratory settings using cells or tissues. It is important to note that these products are not categorized as medicines or drugs, and they have not received approval from the FDA for the prevention, treatment, or cure of any medical condition, ailment, or disease. We must emphasize that any form of bodily introduction of these products into humans or animals is strictly prohibited by law. It is essential to adhere to these guidelines to ensure compliance with legal and ethical standards in research and experimentation.