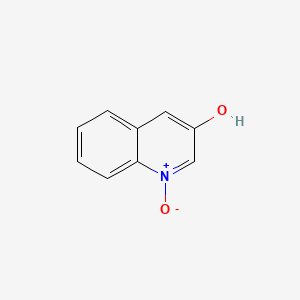
3-Hydroxyquinoline N-oxide
Overview
Description
3-Hydroxyquinoline N-oxide is a derivative of quinoline, a nitrogen-based heterocyclic aromatic compound
Mechanism of Action
Target of Action
3-Hydroxyquinoline N-oxide is a functionalized quinoline, a structural unit that is widespread in various natural products and functional materials . It has been found to target mitochondrial complex II (succinate-ubiquinone oxidoreductase) . This complex plays a crucial role in the electron transport chain, which is essential for cellular respiration and energy production.
Mode of Action
The compound acts as an inhibitor of mitochondrial complex II . By inhibiting this complex, it disrupts the normal flow of electrons within the electron transport chain, leading to a decrease in the production of ATP, the main energy currency of the cell. This disruption can lead to cell death, making this compound a potential therapeutic agent.
Biochemical Pathways
The inhibition of mitochondrial complex II by this compound affects the electron transport chain, a key biochemical pathway in cellular respiration . This disruption can lead to a decrease in ATP production, affecting various downstream cellular processes that rely on ATP, such as signal transduction, DNA replication, and muscle contraction.
Pharmacokinetics
The compound is synthesized from ketones having a 2-nitrophenyl group at the α-position relative to the carbonyl group . The substrates are prepared via a S_NAr reaction or a Sonogashira coupling
Result of Action
The primary result of this compound’s action is the inhibition of mitochondrial complex II, leading to a disruption in the electron transport chain . This disruption decreases ATP production, which can lead to cell death. Therefore, this compound has potential therapeutic applications, particularly in conditions where the induction of cell death is beneficial, such as in cancer treatment.
Biochemical Analysis
Biochemical Properties
3-Hydroxyquinoline N-oxide interacts with various enzymes and proteins in biochemical reactions. It is known to inhibit the succinate-ubiquinone reductase activity of mitochondrial complex II . This interaction is crucial in the electron transport chain, a key biochemical pathway involved in energy production.
Cellular Effects
The effects of this compound on cells are primarily related to its impact on mitochondrial function. By inhibiting complex II, it disrupts the electron transport chain, potentially affecting ATP production and overall cellular metabolism . This can influence cell signaling pathways and gene expression, altering cell function .
Molecular Mechanism
The molecular mechanism of this compound involves its binding to complex II in the mitochondria. This binding inhibits the enzyme’s activity, disrupting the flow of electrons in the electron transport chain . This can lead to changes in gene expression and cellular metabolism.
Temporal Effects in Laboratory Settings
Over time, the effects of this compound can change. For instance, its inhibitory effect on complex II may lead to compensatory changes in other parts of the electron transport chain . Information on the product’s stability, degradation, and long-term effects on cellular function in in vitro or in vivo studies is currently limited.
Dosage Effects in Animal Models
The effects of this compound can vary with dosage in animal models. While specific studies on this compound are limited, related compounds have shown dose-dependent effects . High doses could potentially lead to toxic or adverse effects due to the disruption of mitochondrial function.
Metabolic Pathways
This compound is involved in the electron transport chain, a key metabolic pathway in cells. It interacts with complex II, a component of this pathway, and can affect the flow of electrons, which in turn influences metabolic flux and metabolite levels .
Subcellular Localization
The subcellular localization of this compound is likely within the mitochondria, given its interaction with complex II, a mitochondrial enzyme . Its localization could affect its activity or function, potentially influencing cellular metabolism and energy production.
Preparation Methods
Synthetic Routes and Reaction Conditions
3-Hydroxyquinoline N-oxide can be synthesized from ketones having a 2-nitrophenyl group at the α-position relative to the carbonyl group. The substrates are prepared via a nucleophilic aromatic substitution reaction or a Sonogashira coupling. Treatment with sodium tert-butoxide in dimethyl sulfoxide produces the corresponding quinoline N-oxides .
Another method involves the oxidation of 3,4-dihydroquinoline with oxygen. The general procedure includes extracting the compound with dichloromethane, washing with a saturated aqueous solution of sodium bicarbonate, and drying over magnesium sulfate .
Industrial Production Methods
Industrial production methods for this compound typically involve large-scale synthesis using the aforementioned synthetic routes. The process is optimized for yield and purity, ensuring that the compound meets industrial standards for various applications.
Chemical Reactions Analysis
Types of Reactions
3-Hydroxyquinoline N-oxide undergoes various chemical reactions, including:
Oxidation: The compound can be oxidized to form quinoline derivatives.
Reduction: Reduction reactions can convert the N-oxide group back to the parent quinoline.
Substitution: Electrophilic and nucleophilic substitution reactions are common due to the presence of the nitrogen atom in the quinoline ring.
Common Reagents and Conditions
Oxidation: Oxygen or other oxidizing agents.
Reduction: Reducing agents such as sodium borohydride.
Substitution: Various electrophiles and nucleophiles under appropriate conditions.
Major Products Formed
The major products formed from these reactions include various quinoline derivatives, which can be further functionalized for specific applications .
Scientific Research Applications
Anticancer Activity
Recent studies have highlighted the potential of 3-hydroxyquinoline N-oxide as an anticancer agent. The compound exhibits cytotoxic effects against various cancer cell lines, including those resistant to conventional therapies. Its mechanism involves the induction of apoptosis and inhibition of tumor growth through modulation of specific signaling pathways.
Case Study:
A study demonstrated that derivatives of this compound showed enhanced activity against multidrug-resistant (MDR) cancer cells compared to their non-modified counterparts. This suggests that structural modifications can improve therapeutic efficacy against challenging cancer types .
Antibacterial Properties
This compound has also been investigated for its antibacterial properties. The compound displays significant activity against a range of bacterial pathogens, including those responsible for nosocomial infections.
Data Table: Antibacterial Activity
Bacterial Strain | Minimum Inhibitory Concentration (MIC) | Reference |
---|---|---|
Staphylococcus aureus | 16 µg/mL | |
Escherichia coli | 32 µg/mL | |
Pseudomonas aeruginosa | 64 µg/mL |
This table summarizes the MIC values for various bacterial strains, indicating the effectiveness of this compound as an antibacterial agent.
Neuroprotective Effects
The neuroprotective effects of this compound have been explored in models of neurodegenerative diseases. The compound has shown promise in protecting neuronal cells from oxidative stress and apoptosis.
Case Study:
In a model of Alzheimer's disease, treatment with this compound resulted in reduced levels of amyloid-beta plaques and improved cognitive function in treated animals compared to controls .
Synthesis and Chemical Properties
The synthesis of this compound typically involves oxidation reactions starting from quinoline derivatives. Various methods have been developed to achieve high yields and purity suitable for pharmaceutical applications.
Synthesis Method:
A common method involves the oxidation of 3-hydroxyquinoline using oxidizing agents such as hydrogen peroxide or lead tetraacetate .
Comparison with Similar Compounds
Similar Compounds
8-Hydroxyquinoline: Another hydroxylated quinoline derivative with similar applications in chemistry and biology.
2-Heptyl-4-hydroxyquinoline N-oxide: Known for its antibacterial properties and mechanism of action.
Uniqueness
3-Hydroxyquinoline N-oxide is unique due to its specific substitution pattern, which imparts distinct chemical and biological properties. Its ability to undergo various chemical reactions and form stable complexes with metal ions makes it a valuable compound for research and industrial applications .
Biological Activity
3-Hydroxyquinoline N-oxide (HQNO) is a compound of significant interest in biological and medicinal chemistry due to its diverse biological activities. This article provides an overview of the biological activity of HQNO, highlighting its antimicrobial, anticancer, and other pharmacological properties supported by various research findings.
Chemical Structure and Properties
This compound is derived from quinoline, where the hydroxyl group is positioned at the 3rd carbon of the quinoline ring, and an N-oxide functional group is attached to the nitrogen atom. This structure contributes to its unique reactivity and biological properties.
1. Antimicrobial Activity
HQNO has demonstrated potent antimicrobial properties against various bacterial strains. Research indicates that it inhibits the growth of Gram-positive bacteria more effectively than Gram-negative strains. For instance, studies have shown that HQNO exhibits significant inhibition zones against Staphylococcus aureus and Bacillus subtilis .
Bacterial Strain | Inhibition Zone (mm) |
---|---|
Staphylococcus aureus | 22 |
Bacillus subtilis | 23 |
Klebsiella pneumoniae | 25 |
2. Anticancer Activity
Recent studies have explored the anticancer potential of HQNO derivatives. For example, compounds derived from HQNO have shown cytotoxic effects against various cancer cell lines, including MCF-7 (breast cancer) and KB-V1 (cervical cancer). The mechanism of action appears to involve interference with tubulin polymerization and reactive oxygen species (ROS) generation, leading to apoptosis in cancer cells .
Case Study:
A derivative of HQNO exhibited an IC50 value of 20 nM against MCF-7 cells, indicating strong anticancer activity compared to standard chemotherapy agents .
3. Quorum Sensing Inhibition
HQNO plays a role in quorum sensing among bacteria, particularly in Pseudomonas aeruginosa. It acts as a signaling molecule that influences bacterial communication and virulence. The compound's ability to modulate quorum sensing pathways suggests potential applications in managing bacterial infections by disrupting these communication networks .
Metabolism and Toxicity
The metabolism of HQNO involves its conversion into various conjugated forms, primarily glucuronides and sulfates, which are excreted through urine. Studies indicate that less than 5% of administered HQNO is excreted unchanged, highlighting its extensive metabolic processing . Toxicity studies have shown that high doses can lead to liver tumors in animal models, necessitating caution in therapeutic applications .
Properties
IUPAC Name |
1-oxidoquinolin-1-ium-3-ol | |
---|---|---|
Source | PubChem | |
URL | https://pubchem.ncbi.nlm.nih.gov | |
Description | Data deposited in or computed by PubChem | |
InChI |
InChI=1S/C9H7NO2/c11-8-5-7-3-1-2-4-9(7)10(12)6-8/h1-6,11H | |
Source | PubChem | |
URL | https://pubchem.ncbi.nlm.nih.gov | |
Description | Data deposited in or computed by PubChem | |
InChI Key |
WXOCAKHNPIOFBW-UHFFFAOYSA-N | |
Source | PubChem | |
URL | https://pubchem.ncbi.nlm.nih.gov | |
Description | Data deposited in or computed by PubChem | |
Canonical SMILES |
C1=CC=C2C(=C1)C=C(C=[N+]2[O-])O | |
Source | PubChem | |
URL | https://pubchem.ncbi.nlm.nih.gov | |
Description | Data deposited in or computed by PubChem | |
Molecular Formula |
C9H7NO2 | |
Source | PubChem | |
URL | https://pubchem.ncbi.nlm.nih.gov | |
Description | Data deposited in or computed by PubChem | |
DSSTOX Substance ID |
DTXSID80344232 | |
Record name | 3-Hydroxyquinoline N-oxide | |
Source | EPA DSSTox | |
URL | https://comptox.epa.gov/dashboard/DTXSID80344232 | |
Description | DSSTox provides a high quality public chemistry resource for supporting improved predictive toxicology. | |
Molecular Weight |
161.16 g/mol | |
Source | PubChem | |
URL | https://pubchem.ncbi.nlm.nih.gov | |
Description | Data deposited in or computed by PubChem | |
CAS No. |
59953-98-9 | |
Record name | 3-Hydroxyquinoline N-oxide | |
Source | EPA DSSTox | |
URL | https://comptox.epa.gov/dashboard/DTXSID80344232 | |
Description | DSSTox provides a high quality public chemistry resource for supporting improved predictive toxicology. | |
Retrosynthesis Analysis
AI-Powered Synthesis Planning: Our tool employs the Template_relevance Pistachio, Template_relevance Bkms_metabolic, Template_relevance Pistachio_ringbreaker, Template_relevance Reaxys, Template_relevance Reaxys_biocatalysis model, leveraging a vast database of chemical reactions to predict feasible synthetic routes.
One-Step Synthesis Focus: Specifically designed for one-step synthesis, it provides concise and direct routes for your target compounds, streamlining the synthesis process.
Accurate Predictions: Utilizing the extensive PISTACHIO, BKMS_METABOLIC, PISTACHIO_RINGBREAKER, REAXYS, REAXYS_BIOCATALYSIS database, our tool offers high-accuracy predictions, reflecting the latest in chemical research and data.
Strategy Settings
Precursor scoring | Relevance Heuristic |
---|---|
Min. plausibility | 0.01 |
Model | Template_relevance |
Template Set | Pistachio/Bkms_metabolic/Pistachio_ringbreaker/Reaxys/Reaxys_biocatalysis |
Top-N result to add to graph | 6 |
Feasible Synthetic Routes
Disclaimer and Information on In-Vitro Research Products
Please be aware that all articles and product information presented on BenchChem are intended solely for informational purposes. The products available for purchase on BenchChem are specifically designed for in-vitro studies, which are conducted outside of living organisms. In-vitro studies, derived from the Latin term "in glass," involve experiments performed in controlled laboratory settings using cells or tissues. It is important to note that these products are not categorized as medicines or drugs, and they have not received approval from the FDA for the prevention, treatment, or cure of any medical condition, ailment, or disease. We must emphasize that any form of bodily introduction of these products into humans or animals is strictly prohibited by law. It is essential to adhere to these guidelines to ensure compliance with legal and ethical standards in research and experimentation.