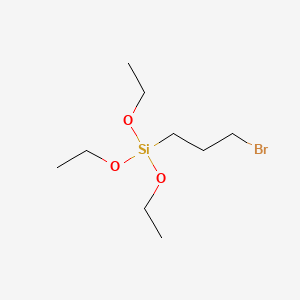
(3-Bromopropyl)triethoxysilane
Overview
Description
(3-Bromopropyl)triethoxysilane is an organosilicon compound with the molecular formula C9H21BrO3Si. It is a colorless to light yellow liquid that is commonly used as a silane coupling agent. This compound is known for its ability to form strong bonds with both organic and inorganic materials, making it valuable in various industrial and research applications.
Preparation Methods
Synthetic Routes and Reaction Conditions: (3-Bromopropyl)triethoxysilane can be synthesized through the reaction of (3-Bromopropyl)trichlorosilane with ethanol in the presence of a base such as pyridine. The reaction typically proceeds as follows:
(3-Bromopropyl)trichlorosilane+3C2H5OH→this compound+3HCl
The reaction is carried out under anhydrous conditions to prevent hydrolysis of the silane.
Industrial Production Methods: Industrial production of this compound involves similar synthetic routes but on a larger scale. The process is optimized for high yield and purity, often using continuous flow reactors and advanced purification techniques to ensure the removal of impurities.
Types of Reactions:
Substitution Reactions: this compound undergoes nucleophilic substitution reactions where the bromine atom is replaced by various nucleophiles such as amines, thiols, or alcohols.
Hydrolysis and Condensation: In the presence of water, this compound hydrolyzes to form silanols, which can further condense to form siloxane bonds.
Common Reagents and Conditions:
Nucleophiles: Amines, thiols, alcohols
Solvents: Anhydrous solvents such as tetrahydrofuran (THF) or dichloromethane (DCM)
Catalysts: Bases like pyridine or triethylamine
Major Products:
Substituted Silanes: Products where the bromine atom is replaced by the nucleophile
Siloxanes: Formed through hydrolysis and condensation reactions
Scientific Research Applications
(3-Bromopropyl)triethoxysilane is widely used in scientific research due to its versatility:
Chemistry: Used as a precursor for the synthesis of various organosilicon compounds and as a coupling agent to enhance the adhesion of organic materials to inorganic surfaces.
Biology: Employed in the functionalization of nanoparticles for targeted drug delivery and imaging applications.
Medicine: Utilized in the development of biocompatible coatings for medical devices and implants.
Industry: Applied in the production of advanced materials such as polymer composites, adhesives, and sealants.
Mechanism of Action
The primary mechanism of action of (3-Bromopropyl)triethoxysilane involves the formation of covalent bonds with both organic and inorganic substrates. The ethoxy groups hydrolyze in the presence of moisture to form silanols, which can then condense with other silanols or hydroxyl groups on surfaces to form stable siloxane bonds. This property makes it an effective coupling agent, enhancing the mechanical and chemical properties of composite materials.
Comparison with Similar Compounds
- (3-Chloropropyl)triethoxysilane
- (3-Iodopropyl)triethoxysilane
- (3-Aminopropyl)triethoxysilane
- (3-Mercaptopropyl)triethoxysilane
Comparison:
- (3-Chloropropyl)triethoxysilane: Similar in structure but with a chlorine atom instead of bromine. It is less reactive due to the lower leaving group ability of chlorine compared to bromine.
- (3-Iodopropyl)triethoxysilane: Contains an iodine atom, making it more reactive than (3-Bromopropyl)triethoxysilane due to the better leaving group ability of iodine.
- (3-Aminopropyl)triethoxysilane: Contains an amino group, making it useful for introducing amine functionalities to surfaces.
- (3-Mercaptopropyl)triethoxysilane: Contains a thiol group, which is useful for binding to metals and forming strong metal-sulfur bonds.
This compound stands out due to its balanced reactivity and versatility, making it suitable for a wide range of applications in both research and industry.
Properties
IUPAC Name |
3-bromopropyl(triethoxy)silane | |
---|---|---|
Source | PubChem | |
URL | https://pubchem.ncbi.nlm.nih.gov | |
Description | Data deposited in or computed by PubChem | |
InChI |
InChI=1S/C9H21BrO3Si/c1-4-11-14(12-5-2,13-6-3)9-7-8-10/h4-9H2,1-3H3 | |
Source | PubChem | |
URL | https://pubchem.ncbi.nlm.nih.gov | |
Description | Data deposited in or computed by PubChem | |
InChI Key |
JMFBXUMHVSZUKY-UHFFFAOYSA-N | |
Source | PubChem | |
URL | https://pubchem.ncbi.nlm.nih.gov | |
Description | Data deposited in or computed by PubChem | |
Canonical SMILES |
CCO[Si](CCCBr)(OCC)OCC | |
Source | PubChem | |
URL | https://pubchem.ncbi.nlm.nih.gov | |
Description | Data deposited in or computed by PubChem | |
Molecular Formula |
C9H21BrO3Si | |
Source | PubChem | |
URL | https://pubchem.ncbi.nlm.nih.gov | |
Description | Data deposited in or computed by PubChem | |
DSSTOX Substance ID |
DTXSID10200079 | |
Record name | (3-Bromopropyl)triethoxysilane | |
Source | EPA DSSTox | |
URL | https://comptox.epa.gov/dashboard/DTXSID10200079 | |
Description | DSSTox provides a high quality public chemistry resource for supporting improved predictive toxicology. | |
Molecular Weight |
285.25 g/mol | |
Source | PubChem | |
URL | https://pubchem.ncbi.nlm.nih.gov | |
Description | Data deposited in or computed by PubChem | |
CAS No. |
52090-18-3 | |
Record name | (3-Bromopropyl)triethoxysilane | |
Source | CAS Common Chemistry | |
URL | https://commonchemistry.cas.org/detail?cas_rn=52090-18-3 | |
Description | CAS Common Chemistry is an open community resource for accessing chemical information. Nearly 500,000 chemical substances from CAS REGISTRY cover areas of community interest, including common and frequently regulated chemicals, and those relevant to high school and undergraduate chemistry classes. This chemical information, curated by our expert scientists, is provided in alignment with our mission as a division of the American Chemical Society. | |
Explanation | The data from CAS Common Chemistry is provided under a CC-BY-NC 4.0 license, unless otherwise stated. | |
Record name | (3-Bromopropyl)triethoxysilane | |
Source | ChemIDplus | |
URL | https://pubchem.ncbi.nlm.nih.gov/substance/?source=chemidplus&sourceid=0052090183 | |
Description | ChemIDplus is a free, web search system that provides access to the structure and nomenclature authority files used for the identification of chemical substances cited in National Library of Medicine (NLM) databases, including the TOXNET system. | |
Record name | (3-Bromopropyl)triethoxysilane | |
Source | EPA DSSTox | |
URL | https://comptox.epa.gov/dashboard/DTXSID10200079 | |
Description | DSSTox provides a high quality public chemistry resource for supporting improved predictive toxicology. | |
Record name | (3-bromopropyl)triethoxysilane | |
Source | European Chemicals Agency (ECHA) | |
URL | https://echa.europa.eu/substance-information/-/substanceinfo/100.052.398 | |
Description | The European Chemicals Agency (ECHA) is an agency of the European Union which is the driving force among regulatory authorities in implementing the EU's groundbreaking chemicals legislation for the benefit of human health and the environment as well as for innovation and competitiveness. | |
Explanation | Use of the information, documents and data from the ECHA website is subject to the terms and conditions of this Legal Notice, and subject to other binding limitations provided for under applicable law, the information, documents and data made available on the ECHA website may be reproduced, distributed and/or used, totally or in part, for non-commercial purposes provided that ECHA is acknowledged as the source: "Source: European Chemicals Agency, http://echa.europa.eu/". Such acknowledgement must be included in each copy of the material. ECHA permits and encourages organisations and individuals to create links to the ECHA website under the following cumulative conditions: Links can only be made to webpages that provide a link to the Legal Notice page. | |
Retrosynthesis Analysis
AI-Powered Synthesis Planning: Our tool employs the Template_relevance Pistachio, Template_relevance Bkms_metabolic, Template_relevance Pistachio_ringbreaker, Template_relevance Reaxys, Template_relevance Reaxys_biocatalysis model, leveraging a vast database of chemical reactions to predict feasible synthetic routes.
One-Step Synthesis Focus: Specifically designed for one-step synthesis, it provides concise and direct routes for your target compounds, streamlining the synthesis process.
Accurate Predictions: Utilizing the extensive PISTACHIO, BKMS_METABOLIC, PISTACHIO_RINGBREAKER, REAXYS, REAXYS_BIOCATALYSIS database, our tool offers high-accuracy predictions, reflecting the latest in chemical research and data.
Strategy Settings
Precursor scoring | Relevance Heuristic |
---|---|
Min. plausibility | 0.01 |
Model | Template_relevance |
Template Set | Pistachio/Bkms_metabolic/Pistachio_ringbreaker/Reaxys/Reaxys_biocatalysis |
Top-N result to add to graph | 6 |
Feasible Synthetic Routes
Disclaimer and Information on In-Vitro Research Products
Please be aware that all articles and product information presented on BenchChem are intended solely for informational purposes. The products available for purchase on BenchChem are specifically designed for in-vitro studies, which are conducted outside of living organisms. In-vitro studies, derived from the Latin term "in glass," involve experiments performed in controlled laboratory settings using cells or tissues. It is important to note that these products are not categorized as medicines or drugs, and they have not received approval from the FDA for the prevention, treatment, or cure of any medical condition, ailment, or disease. We must emphasize that any form of bodily introduction of these products into humans or animals is strictly prohibited by law. It is essential to adhere to these guidelines to ensure compliance with legal and ethical standards in research and experimentation.