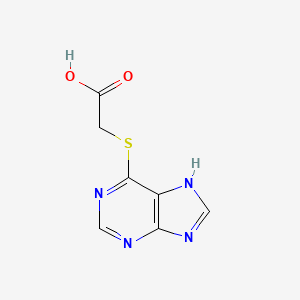
(9H-Purin-6-ylsulfanyl)-acetic acid
Overview
Description
(9H-Purin-6-ylsulfanyl)-acetic acid is a chemical compound that belongs to the purine family Purines are heterocyclic aromatic organic compounds, consisting of a pyrimidine ring fused to an imidazole ring This compound is characterized by the presence of a purine moiety linked to an acetic acid group via a sulfanyl (thioether) linkage
Preparation Methods
Synthetic Routes and Reaction Conditions
The synthesis of (9H-Purin-6-ylsulfanyl)-acetic acid typically involves the reaction of purine derivatives with thiol-containing compounds. One common method is the nucleophilic substitution reaction where a purine derivative, such as 6-chloropurine, reacts with thioglycolic acid under basic conditions to form the desired product. The reaction is usually carried out in a polar aprotic solvent like dimethyl sulfoxide (DMSO) at elevated temperatures to facilitate the nucleophilic attack.
Industrial Production Methods
Industrial production of this compound may involve similar synthetic routes but on a larger scale. The process would be optimized for yield and purity, often involving continuous flow reactors and automated systems to ensure consistent production. The use of catalysts and advanced purification techniques like crystallization and chromatography would be employed to achieve high-quality product suitable for various applications.
Chemical Reactions Analysis
Types of Reactions
(9H-Purin-6-ylsulfanyl)-acetic acid can undergo several types of chemical reactions, including:
Oxidation: The sulfanyl group can be oxidized to form sulfoxides or sulfones.
Reduction: The compound can be reduced to remove the sulfanyl group, yielding purine derivatives.
Substitution: The acetic acid moiety can participate in esterification or amidation reactions.
Common Reagents and Conditions
Oxidation: Reagents like hydrogen peroxide or m-chloroperbenzoic acid (m-CPBA) are commonly used.
Reduction: Reducing agents such as lithium aluminum hydride (LiAlH4) or sodium borohydride (NaBH4) are employed.
Substitution: Acid chlorides or anhydrides are used for esterification, while amines are used for amidation.
Major Products
Oxidation: Sulfoxides and sulfones.
Reduction: Purine derivatives without the sulfanyl group.
Substitution: Esters and amides of this compound.
Scientific Research Applications
(9H-Purin-6-ylsulfanyl)-acetic acid has a wide range of applications in scientific research:
Chemistry: Used as a building block in the synthesis of more complex molecules.
Biology: Studied for its potential role in biochemical pathways involving purines.
Medicine: Investigated for its potential therapeutic properties, including anti-inflammatory and anticancer activities.
Industry: Utilized in the development of novel materials and as a precursor in the synthesis of pharmaceuticals.
Mechanism of Action
The mechanism of action of (9H-Purin-6-ylsulfanyl)-acetic acid involves its interaction with specific molecular targets. The purine moiety can interact with enzymes and receptors involved in nucleotide metabolism, potentially inhibiting or modulating their activity. The sulfanyl group may enhance the compound’s binding affinity and specificity towards these targets, leading to its biological effects.
Comparison with Similar Compounds
Similar Compounds
6-Mercaptopurine: A purine analog used in the treatment of leukemia.
Thioguanine: Another purine analog with anticancer properties.
Allopurinol: A purine derivative used to treat gout by inhibiting xanthine oxidase.
Uniqueness
(9H-Purin-6-ylsulfanyl)-acetic acid is unique due to its specific structural features, particularly the sulfanyl-acetic acid linkage. This structural motif may confer distinct chemical and biological properties compared to other purine derivatives, making it a valuable compound for research and potential therapeutic applications.
Properties
IUPAC Name |
2-(7H-purin-6-ylsulfanyl)acetic acid | |
---|---|---|
Source | PubChem | |
URL | https://pubchem.ncbi.nlm.nih.gov | |
Description | Data deposited in or computed by PubChem | |
InChI |
InChI=1S/C7H6N4O2S/c12-4(13)1-14-7-5-6(9-2-8-5)10-3-11-7/h2-3H,1H2,(H,12,13)(H,8,9,10,11) | |
Source | PubChem | |
URL | https://pubchem.ncbi.nlm.nih.gov | |
Description | Data deposited in or computed by PubChem | |
InChI Key |
YEAVVSQWYLAGNC-UHFFFAOYSA-N | |
Source | PubChem | |
URL | https://pubchem.ncbi.nlm.nih.gov | |
Description | Data deposited in or computed by PubChem | |
Canonical SMILES |
C1=NC2=C(N1)C(=NC=N2)SCC(=O)O | |
Source | PubChem | |
URL | https://pubchem.ncbi.nlm.nih.gov | |
Description | Data deposited in or computed by PubChem | |
Molecular Formula |
C7H6N4O2S | |
Source | PubChem | |
URL | https://pubchem.ncbi.nlm.nih.gov | |
Description | Data deposited in or computed by PubChem | |
DSSTOX Substance ID |
DTXSID50352187 | |
Record name | (9H-Purin-6-ylsulfanyl)-acetic acid | |
Source | EPA DSSTox | |
URL | https://comptox.epa.gov/dashboard/DTXSID50352187 | |
Description | DSSTox provides a high quality public chemistry resource for supporting improved predictive toxicology. | |
Molecular Weight |
210.22 g/mol | |
Source | PubChem | |
URL | https://pubchem.ncbi.nlm.nih.gov | |
Description | Data deposited in or computed by PubChem | |
CAS No. |
608-09-3 | |
Record name | NSC20090 | |
Source | DTP/NCI | |
URL | https://dtp.cancer.gov/dtpstandard/servlet/dwindex?searchtype=NSC&outputformat=html&searchlist=20090 | |
Description | The NCI Development Therapeutics Program (DTP) provides services and resources to the academic and private-sector research communities worldwide to facilitate the discovery and development of new cancer therapeutic agents. | |
Explanation | Unless otherwise indicated, all text within NCI products is free of copyright and may be reused without our permission. Credit the National Cancer Institute as the source. | |
Record name | (9H-Purin-6-ylsulfanyl)-acetic acid | |
Source | EPA DSSTox | |
URL | https://comptox.epa.gov/dashboard/DTXSID50352187 | |
Description | DSSTox provides a high quality public chemistry resource for supporting improved predictive toxicology. | |
Retrosynthesis Analysis
AI-Powered Synthesis Planning: Our tool employs the Template_relevance Pistachio, Template_relevance Bkms_metabolic, Template_relevance Pistachio_ringbreaker, Template_relevance Reaxys, Template_relevance Reaxys_biocatalysis model, leveraging a vast database of chemical reactions to predict feasible synthetic routes.
One-Step Synthesis Focus: Specifically designed for one-step synthesis, it provides concise and direct routes for your target compounds, streamlining the synthesis process.
Accurate Predictions: Utilizing the extensive PISTACHIO, BKMS_METABOLIC, PISTACHIO_RINGBREAKER, REAXYS, REAXYS_BIOCATALYSIS database, our tool offers high-accuracy predictions, reflecting the latest in chemical research and data.
Strategy Settings
Precursor scoring | Relevance Heuristic |
---|---|
Min. plausibility | 0.01 |
Model | Template_relevance |
Template Set | Pistachio/Bkms_metabolic/Pistachio_ringbreaker/Reaxys/Reaxys_biocatalysis |
Top-N result to add to graph | 6 |
Feasible Synthetic Routes
Disclaimer and Information on In-Vitro Research Products
Please be aware that all articles and product information presented on BenchChem are intended solely for informational purposes. The products available for purchase on BenchChem are specifically designed for in-vitro studies, which are conducted outside of living organisms. In-vitro studies, derived from the Latin term "in glass," involve experiments performed in controlled laboratory settings using cells or tissues. It is important to note that these products are not categorized as medicines or drugs, and they have not received approval from the FDA for the prevention, treatment, or cure of any medical condition, ailment, or disease. We must emphasize that any form of bodily introduction of these products into humans or animals is strictly prohibited by law. It is essential to adhere to these guidelines to ensure compliance with legal and ethical standards in research and experimentation.