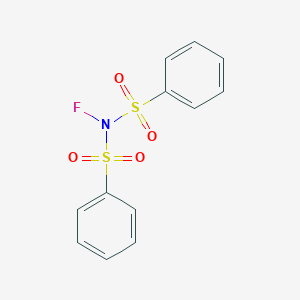
N-Fluorobenzenesulfonimide
Overview
Description
N-Fluorobenzenesulfonimide is a versatile and widely used reagent in organic synthesis. It is primarily employed for the direct fluorination and amination of aromatic and heteroaromatic compounds. This compound is known for its stability, mildness, and high solubility, making it a popular choice in various chemical transformations .
Mechanism of Action
Target of Action
N-Fluorobenzenesulfonimide (NFSI) primarily targets (hetero)aromatic C–H bonds . It is used as a reagent for the direct fluorination and amination of these bonds . The compound’s role is to introduce fluorine into neutral organic molecules and to fluorinate nucleophilic substrates such as reactive organometallic species and malonate anions .
Mode of Action
The interaction of NFSI with its targets involves several steps . First, there is an oxidative addition of Pd(0) to the N–F bond of NFSI to form an intermediate . This is followed by coordination of the Pd(II) complex to the anilide to provide another intermediate . Electrophilic palladation of this intermediate results in a dearomatized spiro-palladacycle intermediate . Amination of this intermediate with –N(SO2Ph)2 forms another intermediate, and finally, hydrogen elimination from this intermediate gives the target product .
Biochemical Pathways
NFSI affects the biochemical pathways involving the direct fluorination and amination of (hetero)aromatic C–H bonds . The downstream effects include the formation of fluoroarenes, which are important structural motifs in medicinal chemistry . These fluoroarenes display a broad spectrum of biological and pharmacological activities, including anticancer, antibacterial, antidepressant, anticonvulsant, antipsychotic, and antiemetic activities .
Pharmacokinetics
It is known that nfsi is a mild, stable, and highly soluble crystalline solid , which suggests that it may have good bioavailability.
Result of Action
The molecular and cellular effects of NFSI’s action include the formation of fluoroarenes . These compounds have various biological and pharmacological activities, as mentioned above . In addition, NFSI can also be used as an amination reagent in various transformations of aliphatic and aromatic compounds .
Action Environment
The action, efficacy, and stability of NFSI can be influenced by environmental factors such as temperature . For example, direct C–H amination of a diverse range of arenes, including N- and S-heteroarenes, with NFSI can be achieved at or below room temperature . The presence of a catalyst, such as a Pd(II) complex, and a co-catalyst, such as Ag(bipy)2ClO4, in MeCN, is also necessary for the reaction .
Biochemical Analysis
Biochemical Properties
N-Fluorobenzenesulfonimide plays a crucial role in biochemical reactions as an electrophilic fluorinating agentFor instance, it has been used in palladium-catalyzed enantioselective fluorination reactions, where it interacts with palladium complexes to achieve high selectivity and yield . Additionally, this compound can fluorinate nucleophilic substrates such as reactive organometallic species and malonate anions . These interactions are essential for the synthesis of fluorinated compounds, which are valuable in pharmaceutical and agrochemical industries.
Cellular Effects
This compound influences various cellular processes by modifying the chemical structure of biomolecules. It can affect cell signaling pathways, gene expression, and cellular metabolism by introducing fluorine atoms into key biomolecules. For example, the fluorination of aromatic compounds can alter their biological activity, leading to changes in cell function . The compound’s ability to modify proteins and enzymes can impact cellular processes such as signal transduction and metabolic pathways, ultimately affecting cell behavior and function.
Molecular Mechanism
The molecular mechanism of this compound involves its role as an electrophilic fluorinating agent. It exerts its effects by forming covalent bonds with nucleophilic sites on biomolecules. The process typically involves the oxidative addition of palladium to the N-F bond of this compound, followed by coordination with the target substrate . This interaction facilitates the transfer of the fluorine atom to the substrate, resulting in the formation of fluorinated products. The compound can also act as a strong oxidant, promoting reductive elimination from transition metals and enabling various chemical transformations .
Temporal Effects in Laboratory Settings
In laboratory settings, the effects of this compound can change over time due to its stability and degradation properties. The compound is known for its stability under standard storage conditions, which allows for consistent performance in biochemical reactions . Prolonged exposure to light and air can lead to degradation, affecting its reactivity and efficacy. Long-term studies have shown that this compound maintains its fluorinating ability over extended periods, making it a reliable reagent for biochemical research .
Dosage Effects in Animal Models
The effects of this compound vary with different dosages in animal models. At low doses, the compound can effectively fluorinate target biomolecules without causing significant toxicity . At higher doses, it may exhibit toxic or adverse effects, including cellular damage and disruption of metabolic processes. Threshold effects have been observed, where a specific dosage range is required to achieve the desired biochemical modifications without inducing toxicity . These findings highlight the importance of optimizing dosage levels for safe and effective use in biochemical research.
Metabolic Pathways
This compound is involved in various metabolic pathways, primarily through its interactions with enzymes and cofactors. It can influence metabolic flux and metabolite levels by modifying key biomolecules involved in metabolic processes . For example, the compound’s ability to fluorinate aromatic compounds can impact the activity of enzymes involved in aromatic amino acid metabolism. Additionally, this compound can interact with cofactors such as NADH and FADH2, affecting their redox states and influencing cellular energy metabolism .
Transport and Distribution
Within cells and tissues, this compound is transported and distributed through interactions with transporters and binding proteins. These interactions facilitate its localization and accumulation in specific cellular compartments . The compound’s ability to bind to proteins and enzymes can influence its distribution and availability for biochemical reactions. Additionally, this compound’s solubility in various solvents allows for efficient transport across cell membranes, enhancing its accessibility to target biomolecules .
Subcellular Localization
The subcellular localization of this compound is influenced by targeting signals and post-translational modifications. The compound can be directed to specific compartments or organelles within the cell, where it exerts its biochemical effects . For instance, this compound may localize to the mitochondria, where it can interact with mitochondrial enzymes and influence cellular energy metabolism. Additionally, its ability to modify proteins and enzymes can affect their subcellular localization and activity, further impacting cellular function .
Preparation Methods
N-Fluorobenzenesulfonimide can be synthesized through several methods. One common synthetic route involves the reaction of benzenesulfonimide with elemental fluorine or other fluorinating agents under controlled conditions. The reaction typically requires a catalyst and is carried out in an inert atmosphere to prevent unwanted side reactions . Industrial production methods often involve large-scale reactions using specialized equipment to ensure safety and efficiency .
Chemical Reactions Analysis
N-Fluorobenzenesulfonimide undergoes various types of chemical reactions, including:
Fluorination: It is used as an electrophilic fluorinating agent to introduce fluorine atoms into organic molecules.
Major products formed from these reactions include fluorinated and aminated aromatic compounds, which are valuable intermediates in pharmaceuticals and agrochemicals .
Scientific Research Applications
N-Fluorobenzenesulfonimide has a wide range of applications in scientific research:
Comparison with Similar Compounds
N-Fluorobenzenesulfonimide is compared with other electrophilic fluorinating agents such as N-fluoropyridinium salts and xenon difluoride. While these compounds also serve as sources of electrophilic fluorine, this compound is often preferred due to its stability, ease of handling, and mild reaction conditions . Similar compounds include:
- N-fluoropyridinium salts
- Xenon difluoride
- N-fluorobenzenesulfonamide
Each of these compounds has its own advantages and limitations, but this compound stands out for its versatility and broad applicability in various chemical transformations .
Properties
IUPAC Name |
N-(benzenesulfonyl)-N-fluorobenzenesulfonamide | |
---|---|---|
Source | PubChem | |
URL | https://pubchem.ncbi.nlm.nih.gov | |
Description | Data deposited in or computed by PubChem | |
InChI |
InChI=1S/C12H10FNO4S2/c13-14(19(15,16)11-7-3-1-4-8-11)20(17,18)12-9-5-2-6-10-12/h1-10H | |
Source | PubChem | |
URL | https://pubchem.ncbi.nlm.nih.gov | |
Description | Data deposited in or computed by PubChem | |
InChI Key |
RLKHFSNWQCZBDC-UHFFFAOYSA-N | |
Source | PubChem | |
URL | https://pubchem.ncbi.nlm.nih.gov | |
Description | Data deposited in or computed by PubChem | |
Canonical SMILES |
C1=CC=C(C=C1)S(=O)(=O)N(F)S(=O)(=O)C2=CC=CC=C2 | |
Source | PubChem | |
URL | https://pubchem.ncbi.nlm.nih.gov | |
Description | Data deposited in or computed by PubChem | |
Molecular Formula |
C12H10FNO4S2 | |
Source | PubChem | |
URL | https://pubchem.ncbi.nlm.nih.gov | |
Description | Data deposited in or computed by PubChem | |
DSSTOX Substance ID |
DTXSID10343177 | |
Record name | N-Fluorobenzenesulfonimide | |
Source | EPA DSSTox | |
URL | https://comptox.epa.gov/dashboard/DTXSID10343177 | |
Description | DSSTox provides a high quality public chemistry resource for supporting improved predictive toxicology. | |
Molecular Weight |
315.3 g/mol | |
Source | PubChem | |
URL | https://pubchem.ncbi.nlm.nih.gov | |
Description | Data deposited in or computed by PubChem | |
CAS No. |
133745-75-2 | |
Record name | N-Fluorobenzenesulfonimide | |
Source | CAS Common Chemistry | |
URL | https://commonchemistry.cas.org/detail?cas_rn=133745-75-2 | |
Description | CAS Common Chemistry is an open community resource for accessing chemical information. Nearly 500,000 chemical substances from CAS REGISTRY cover areas of community interest, including common and frequently regulated chemicals, and those relevant to high school and undergraduate chemistry classes. This chemical information, curated by our expert scientists, is provided in alignment with our mission as a division of the American Chemical Society. | |
Explanation | The data from CAS Common Chemistry is provided under a CC-BY-NC 4.0 license, unless otherwise stated. | |
Record name | N-Fluorobenzenesulfonimide | |
Source | ChemIDplus | |
URL | https://pubchem.ncbi.nlm.nih.gov/substance/?source=chemidplus&sourceid=0133745752 | |
Description | ChemIDplus is a free, web search system that provides access to the structure and nomenclature authority files used for the identification of chemical substances cited in National Library of Medicine (NLM) databases, including the TOXNET system. | |
Record name | N-Fluorobenzenesulfonimide | |
Source | EPA DSSTox | |
URL | https://comptox.epa.gov/dashboard/DTXSID10343177 | |
Description | DSSTox provides a high quality public chemistry resource for supporting improved predictive toxicology. | |
Record name | Benzenesulfonamide, N-fluoro-N-(phenylsulfonyl) | |
Source | European Chemicals Agency (ECHA) | |
URL | https://echa.europa.eu/substance-information/-/substanceinfo/100.114.480 | |
Description | The European Chemicals Agency (ECHA) is an agency of the European Union which is the driving force among regulatory authorities in implementing the EU's groundbreaking chemicals legislation for the benefit of human health and the environment as well as for innovation and competitiveness. | |
Explanation | Use of the information, documents and data from the ECHA website is subject to the terms and conditions of this Legal Notice, and subject to other binding limitations provided for under applicable law, the information, documents and data made available on the ECHA website may be reproduced, distributed and/or used, totally or in part, for non-commercial purposes provided that ECHA is acknowledged as the source: "Source: European Chemicals Agency, http://echa.europa.eu/". Such acknowledgement must be included in each copy of the material. ECHA permits and encourages organisations and individuals to create links to the ECHA website under the following cumulative conditions: Links can only be made to webpages that provide a link to the Legal Notice page. | |
Record name | N-FLUOROBENZENESULFONIMIDE | |
Source | FDA Global Substance Registration System (GSRS) | |
URL | https://gsrs.ncats.nih.gov/ginas/app/beta/substances/38TGL3A00I | |
Description | The FDA Global Substance Registration System (GSRS) enables the efficient and accurate exchange of information on what substances are in regulated products. Instead of relying on names, which vary across regulatory domains, countries, and regions, the GSRS knowledge base makes it possible for substances to be defined by standardized, scientific descriptions. | |
Explanation | Unless otherwise noted, the contents of the FDA website (www.fda.gov), both text and graphics, are not copyrighted. They are in the public domain and may be republished, reprinted and otherwise used freely by anyone without the need to obtain permission from FDA. Credit to the U.S. Food and Drug Administration as the source is appreciated but not required. | |
Retrosynthesis Analysis
AI-Powered Synthesis Planning: Our tool employs the Template_relevance Pistachio, Template_relevance Bkms_metabolic, Template_relevance Pistachio_ringbreaker, Template_relevance Reaxys, Template_relevance Reaxys_biocatalysis model, leveraging a vast database of chemical reactions to predict feasible synthetic routes.
One-Step Synthesis Focus: Specifically designed for one-step synthesis, it provides concise and direct routes for your target compounds, streamlining the synthesis process.
Accurate Predictions: Utilizing the extensive PISTACHIO, BKMS_METABOLIC, PISTACHIO_RINGBREAKER, REAXYS, REAXYS_BIOCATALYSIS database, our tool offers high-accuracy predictions, reflecting the latest in chemical research and data.
Strategy Settings
Precursor scoring | Relevance Heuristic |
---|---|
Min. plausibility | 0.01 |
Model | Template_relevance |
Template Set | Pistachio/Bkms_metabolic/Pistachio_ringbreaker/Reaxys/Reaxys_biocatalysis |
Top-N result to add to graph | 6 |
Feasible Synthetic Routes
Disclaimer and Information on In-Vitro Research Products
Please be aware that all articles and product information presented on BenchChem are intended solely for informational purposes. The products available for purchase on BenchChem are specifically designed for in-vitro studies, which are conducted outside of living organisms. In-vitro studies, derived from the Latin term "in glass," involve experiments performed in controlled laboratory settings using cells or tissues. It is important to note that these products are not categorized as medicines or drugs, and they have not received approval from the FDA for the prevention, treatment, or cure of any medical condition, ailment, or disease. We must emphasize that any form of bodily introduction of these products into humans or animals is strictly prohibited by law. It is essential to adhere to these guidelines to ensure compliance with legal and ethical standards in research and experimentation.