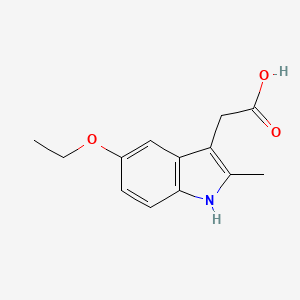
(5-ethoxy-2-methyl-1H-indol-3-yl)acetic acid
Overview
Description
(5-ethoxy-2-methyl-1H-indol-3-yl)acetic acid, also known as 5-EMIA, is a synthetic organic compound with a broad range of applications in the fields of biochemistry, pharmacology, and medicine. 5-EMIA is a derivative of indole-3-acetic acid (IAA), which is a naturally-occurring plant hormone that regulates plant growth and development. 5-EMIA has been widely used in laboratory experiments to study the biochemical and physiological effects of IAA and its derivatives.
Scientific Research Applications
- “(5-ethoxy-2-methyl-1H-indol-3-yl)acetic acid” exhibits anti-cancer activity. Researchers have explored its potential as a chemotherapeutic agent, particularly in inhibiting tumor growth and metastasis. Mechanisms include interference with cell cycle progression and induction of apoptosis in cancer cells .
- The compound demonstrates anti-inflammatory properties. Its hydrazide substitution contributes to this effect, making it a promising candidate for managing inflammatory conditions .
- Studies have investigated the antimicrobial potential of this indole derivative. It shows promise in combating bacterial and fungal infections. Researchers explore its use as an alternative to existing antimicrobial agents .
- “(5-ethoxy-2-methyl-1H-indol-3-yl)acetic acid” may have neuroprotective effects. Researchers have studied its impact on neuronal health, including potential benefits in neurodegenerative diseases .
- Indole derivatives, including indole-3-acetic acid (a related compound), play a crucial role in plant growth regulation. They act as plant hormones, influencing processes like cell elongation, root development, and fruit ripening. “(5-ethoxy-2-methyl-1H-indol-3-yl)acetic acid” might have similar effects .
- Researchers explore the synthesis of novel indole derivatives for drug development. This compound serves as a valuable building block for designing new drugs, especially those targeting specific receptors or pathways .
Anti-Cancer Properties
Anti-Inflammatory Effects
Antimicrobial Activity
Neuroprotective Applications
Plant Growth Regulation
Drug Development and Medicinal Chemistry
Mechanism of Action
Target of Action
It is known that this compound is a derivative of indole-3-acetic acid , which is a type of auxin, a class of plant hormones. Auxins are known to play a crucial role in the coordination of many growth and behavioral processes in plants’ life cycles.
Mode of Action
As an auxin-like compound, it may interact with auxin receptors in plants, triggering a series of events that can lead to changes in gene expression, cell growth, and differentiation .
Biochemical Pathways
These could include cell division, elongation, differentiation, and responses to light and gravity .
Pharmacokinetics
It is soluble in methanol , which suggests it may be absorbed and distributed in organisms that consume it
Result of Action
As a plant growth regulator, it likely influences cell growth and differentiation, potentially leading to changes in plant morphology .
Action Environment
Like other plant growth regulators, its effectiveness may be influenced by various environmental factors such as light, temperature, and moisture .
properties
IUPAC Name |
2-(5-ethoxy-2-methyl-1H-indol-3-yl)acetic acid | |
---|---|---|
Source | PubChem | |
URL | https://pubchem.ncbi.nlm.nih.gov | |
Description | Data deposited in or computed by PubChem | |
InChI |
InChI=1S/C13H15NO3/c1-3-17-9-4-5-12-11(6-9)10(7-13(15)16)8(2)14-12/h4-6,14H,3,7H2,1-2H3,(H,15,16) | |
Source | PubChem | |
URL | https://pubchem.ncbi.nlm.nih.gov | |
Description | Data deposited in or computed by PubChem | |
InChI Key |
UTQVPTYRFOINMB-UHFFFAOYSA-N | |
Source | PubChem | |
URL | https://pubchem.ncbi.nlm.nih.gov | |
Description | Data deposited in or computed by PubChem | |
Canonical SMILES |
CCOC1=CC2=C(C=C1)NC(=C2CC(=O)O)C | |
Source | PubChem | |
URL | https://pubchem.ncbi.nlm.nih.gov | |
Description | Data deposited in or computed by PubChem | |
Molecular Formula |
C13H15NO3 | |
Source | PubChem | |
URL | https://pubchem.ncbi.nlm.nih.gov | |
Description | Data deposited in or computed by PubChem | |
DSSTOX Substance ID |
DTXSID20281753 | |
Record name | (5-ethoxy-2-methyl-1H-indol-3-yl)acetic acid | |
Source | EPA DSSTox | |
URL | https://comptox.epa.gov/dashboard/DTXSID20281753 | |
Description | DSSTox provides a high quality public chemistry resource for supporting improved predictive toxicology. | |
Molecular Weight |
233.26 g/mol | |
Source | PubChem | |
URL | https://pubchem.ncbi.nlm.nih.gov | |
Description | Data deposited in or computed by PubChem | |
Product Name |
(5-ethoxy-2-methyl-1H-indol-3-yl)acetic acid | |
CAS RN |
34024-46-9 | |
Record name | 34024-46-9 | |
Source | DTP/NCI | |
URL | https://dtp.cancer.gov/dtpstandard/servlet/dwindex?searchtype=NSC&outputformat=html&searchlist=22873 | |
Description | The NCI Development Therapeutics Program (DTP) provides services and resources to the academic and private-sector research communities worldwide to facilitate the discovery and development of new cancer therapeutic agents. | |
Explanation | Unless otherwise indicated, all text within NCI products is free of copyright and may be reused without our permission. Credit the National Cancer Institute as the source. | |
Record name | (5-ethoxy-2-methyl-1H-indol-3-yl)acetic acid | |
Source | EPA DSSTox | |
URL | https://comptox.epa.gov/dashboard/DTXSID20281753 | |
Description | DSSTox provides a high quality public chemistry resource for supporting improved predictive toxicology. | |
Retrosynthesis Analysis
AI-Powered Synthesis Planning: Our tool employs the Template_relevance Pistachio, Template_relevance Bkms_metabolic, Template_relevance Pistachio_ringbreaker, Template_relevance Reaxys, Template_relevance Reaxys_biocatalysis model, leveraging a vast database of chemical reactions to predict feasible synthetic routes.
One-Step Synthesis Focus: Specifically designed for one-step synthesis, it provides concise and direct routes for your target compounds, streamlining the synthesis process.
Accurate Predictions: Utilizing the extensive PISTACHIO, BKMS_METABOLIC, PISTACHIO_RINGBREAKER, REAXYS, REAXYS_BIOCATALYSIS database, our tool offers high-accuracy predictions, reflecting the latest in chemical research and data.
Strategy Settings
Precursor scoring | Relevance Heuristic |
---|---|
Min. plausibility | 0.01 |
Model | Template_relevance |
Template Set | Pistachio/Bkms_metabolic/Pistachio_ringbreaker/Reaxys/Reaxys_biocatalysis |
Top-N result to add to graph | 6 |
Feasible Synthetic Routes
Disclaimer and Information on In-Vitro Research Products
Please be aware that all articles and product information presented on BenchChem are intended solely for informational purposes. The products available for purchase on BenchChem are specifically designed for in-vitro studies, which are conducted outside of living organisms. In-vitro studies, derived from the Latin term "in glass," involve experiments performed in controlled laboratory settings using cells or tissues. It is important to note that these products are not categorized as medicines or drugs, and they have not received approval from the FDA for the prevention, treatment, or cure of any medical condition, ailment, or disease. We must emphasize that any form of bodily introduction of these products into humans or animals is strictly prohibited by law. It is essential to adhere to these guidelines to ensure compliance with legal and ethical standards in research and experimentation.