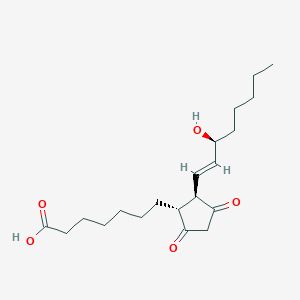
Prostaglandin K1
Overview
Description
Prostaglandin K1 is a bioactive lipid compound derived from arachidonic acid. Prostaglandins are a group of physiologically active lipid compounds having diverse hormone-like effects in animals. They are involved in various physiological processes such as inflammation, blood flow, the formation of blood clots, and the induction of labor.
Mechanism of Action
Target of Action
Prostaglandin K1, like other prostaglandins, primarily targets G-protein-coupled receptors (GPCRs) . These receptors are specific to prostanoids and are associated with a variety of G-proteins .
Biochemical Pathways
Prostaglandins, including this compound, are derived enzymatically from fatty acids . They are involved in a wide range of roles from homeostasis of blood pressure and blood flow, the initiation and resolution of inflammation, the perception of pain, cell survival, and the progression of numerous disease states . These biomolecules act most often as autocrine or paracrine signaling agents and most have relatively short half-lives .
Pharmacokinetics
Prostaglandins in general have been shown to have relatively short half-lives . They are often administered via intra-arterial or intravenous infusions . The metabolism and pharmacokinetics of prostaglandins are complex and can be influenced by various factors, including the method of administration .
Action Environment
The action, efficacy, and stability of this compound can be influenced by various environmental factors. For example, the abundance of aggressive bacteria was found to be positively correlated with the levels of certain prostaglandins . This suggests that the gut microbiota could potentially influence the action of this compound .
Biochemical Analysis
Biochemical Properties
Prostaglandin K1 plays a role in several biochemical reactions. It interacts with enzymes such as cyclooxygenase (COX) and 15-hydroxyprostaglandin dehydrogenase (15-PGDH). Cyclooxygenase is involved in the initial steps of prostaglandin synthesis, converting arachidonic acid to prostaglandin H2, which is then further metabolized to various prostaglandins, including this compound . This compound is resistant to metabolism by 15-hydroxyprostaglandin dehydrogenase in vitro, which suggests it may have a prolonged effect compared to other prostaglandins .
Cellular Effects
This compound influences various cellular processes, including cell signaling pathways, gene expression, and cellular metabolism. It has been shown to affect the function of smooth muscle cells, endothelial cells, and platelets. By acting on the Prostaglandin E2 receptor subtype EP1, this compound can modulate intracellular signaling pathways that regulate inflammation, vasodilation, and platelet aggregation . These effects are crucial in maintaining vascular homeostasis and responding to injury or inflammation.
Molecular Mechanism
At the molecular level, this compound exerts its effects through binding interactions with specific receptors and enzymes. As an agonist of the Prostaglandin E2 receptor subtype EP1, it activates downstream signaling cascades involving G-proteins and second messengers such as cyclic AMP (cAMP). This activation can lead to changes in gene expression and enzyme activity, ultimately influencing cellular responses such as inflammation and vasodilation .
Temporal Effects in Laboratory Settings
In laboratory settings, the effects of this compound can change over time. Its stability and degradation are important factors in determining its long-term effects on cellular function. This compound is known to be resistant to metabolism by 15-hydroxyprostaglandin dehydrogenase, which may contribute to its prolonged activity in vitro . Long-term studies have shown that this compound can maintain its effects on cellular processes such as inflammation and vasodilation over extended periods.
Dosage Effects in Animal Models
The effects of this compound vary with different dosages in animal models. At low doses, it can effectively modulate inflammation and vasodilation without causing significant adverse effects. At high doses, this compound may induce toxic effects, including excessive vasodilation and hypotension . These threshold effects highlight the importance of careful dosage management in therapeutic applications.
Metabolic Pathways
This compound is involved in metabolic pathways related to prostaglandin synthesis and degradation. It is synthesized from arachidonic acid through the action of cyclooxygenase and other enzymes. This compound is resistant to degradation by 15-hydroxyprostaglandin dehydrogenase, which may result in prolonged activity and accumulation in tissues . This resistance to metabolism can influence metabolic flux and the levels of other metabolites in the pathway.
Transport and Distribution
Within cells and tissues, this compound is transported and distributed through interactions with specific transporters and binding proteins. These interactions can affect its localization and accumulation in different cellular compartments. This compound’s resistance to metabolism may also influence its distribution, allowing it to exert prolonged effects in target tissues .
Subcellular Localization
This compound’s subcellular localization is influenced by targeting signals and post-translational modifications. These factors can direct this compound to specific compartments or organelles within the cell, affecting its activity and function. For example, localization to the plasma membrane can facilitate interactions with cell surface receptors, while localization to the nucleus can influence gene expression .
Preparation Methods
Synthetic Routes and Reaction Conditions: Prostaglandin K1 can be synthesized through a chemoenzymatic process. This involves the use of bromohydrin as a radical equivalent of Corey lactone, which is synthesized in two steps. The chiral cyclopentane core is introduced with high enantioselectivity, and the lipid chains are incorporated through bromohydrin formation, nickel-catalyzed cross-couplings, and Wittig reactions .
Industrial Production Methods: Industrial production of prostaglandins typically involves the use of Corey lactone intermediates. The Corey procedure uses δ-lactone and γ-lactone intermediates with stereocenters on the cyclopentane fragment to link the prostaglandin side chains .
Chemical Reactions Analysis
Types of Reactions: Prostaglandin K1 undergoes various types of chemical reactions, including oxidation, reduction, and substitution reactions.
Common Reagents and Conditions:
Oxidation: this compound can be oxidized using reagents such as potassium permanganate or chromium trioxide.
Reduction: Reduction reactions can be carried out using reagents like lithium aluminum hydride or sodium borohydride.
Substitution: Substitution reactions often involve halogenation using reagents like bromine or chlorine.
Major Products Formed: The major products formed from these reactions include various prostaglandin analogs and derivatives, which are used in different therapeutic applications .
Scientific Research Applications
Prostaglandin K1 has a wide range of scientific research applications:
Chemistry: Used as a model compound for studying lipid oxidation and reduction reactions.
Biology: Plays a role in cell signaling and regulation of physiological processes.
Medicine: Used in the treatment of conditions such as glaucoma, pulmonary arterial hypertension, and to induce labor.
Industry: Employed in the synthesis of various prostaglandin analogs used in pharmaceuticals
Comparison with Similar Compounds
- Prostaglandin E1
- Prostaglandin F2α
- Prostaglandin I2
- Thromboxane A2
- Leukotrienes
Comparison: Prostaglandin K1 is unique in its specific receptor interactions and the physiological responses it mediates. While Prostaglandin E1 and Prostaglandin I2 are primarily involved in vasodilation and inhibition of platelet aggregation, Prostaglandin F2α is more associated with uterine contractions. Thromboxane A2 promotes platelet aggregation and vasoconstriction, whereas leukotrienes are involved in inflammatory responses .
This compound’s distinct receptor interactions and signaling pathways make it a valuable compound in both research and therapeutic applications.
Properties
IUPAC Name |
7-[(1R,2R)-2-[(E,3S)-3-hydroxyoct-1-enyl]-3,5-dioxocyclopentyl]heptanoic acid | |
---|---|---|
Source | PubChem | |
URL | https://pubchem.ncbi.nlm.nih.gov | |
Description | Data deposited in or computed by PubChem | |
InChI |
InChI=1S/C20H32O5/c1-2-3-6-9-15(21)12-13-17-16(18(22)14-19(17)23)10-7-4-5-8-11-20(24)25/h12-13,15-17,21H,2-11,14H2,1H3,(H,24,25)/b13-12+/t15-,16+,17+/m0/s1 | |
Source | PubChem | |
URL | https://pubchem.ncbi.nlm.nih.gov | |
Description | Data deposited in or computed by PubChem | |
InChI Key |
KJWZYMMLVHIVSU-IYCNHOCDSA-N | |
Source | PubChem | |
URL | https://pubchem.ncbi.nlm.nih.gov | |
Description | Data deposited in or computed by PubChem | |
Canonical SMILES |
CCCCCC(C=CC1C(C(=O)CC1=O)CCCCCCC(=O)O)O | |
Source | PubChem | |
URL | https://pubchem.ncbi.nlm.nih.gov | |
Description | Data deposited in or computed by PubChem | |
Isomeric SMILES |
CCCCC[C@@H](/C=C/[C@@H]1[C@H](C(=O)CC1=O)CCCCCCC(=O)O)O | |
Source | PubChem | |
URL | https://pubchem.ncbi.nlm.nih.gov | |
Description | Data deposited in or computed by PubChem | |
Molecular Formula |
C20H32O5 | |
Source | PubChem | |
URL | https://pubchem.ncbi.nlm.nih.gov | |
Description | Data deposited in or computed by PubChem | |
DSSTOX Substance ID |
DTXSID401348011 | |
Record name | Prostaglandin K1 | |
Source | EPA DSSTox | |
URL | https://comptox.epa.gov/dashboard/DTXSID401348011 | |
Description | DSSTox provides a high quality public chemistry resource for supporting improved predictive toxicology. | |
Molecular Weight |
352.5 g/mol | |
Source | PubChem | |
URL | https://pubchem.ncbi.nlm.nih.gov | |
Description | Data deposited in or computed by PubChem | |
CAS No. |
69413-73-6 | |
Record name | Prostaglandin K1 | |
Source | EPA DSSTox | |
URL | https://comptox.epa.gov/dashboard/DTXSID401348011 | |
Description | DSSTox provides a high quality public chemistry resource for supporting improved predictive toxicology. | |
Retrosynthesis Analysis
AI-Powered Synthesis Planning: Our tool employs the Template_relevance Pistachio, Template_relevance Bkms_metabolic, Template_relevance Pistachio_ringbreaker, Template_relevance Reaxys, Template_relevance Reaxys_biocatalysis model, leveraging a vast database of chemical reactions to predict feasible synthetic routes.
One-Step Synthesis Focus: Specifically designed for one-step synthesis, it provides concise and direct routes for your target compounds, streamlining the synthesis process.
Accurate Predictions: Utilizing the extensive PISTACHIO, BKMS_METABOLIC, PISTACHIO_RINGBREAKER, REAXYS, REAXYS_BIOCATALYSIS database, our tool offers high-accuracy predictions, reflecting the latest in chemical research and data.
Strategy Settings
Precursor scoring | Relevance Heuristic |
---|---|
Min. plausibility | 0.01 |
Model | Template_relevance |
Template Set | Pistachio/Bkms_metabolic/Pistachio_ringbreaker/Reaxys/Reaxys_biocatalysis |
Top-N result to add to graph | 6 |
Feasible Synthetic Routes
Disclaimer and Information on In-Vitro Research Products
Please be aware that all articles and product information presented on BenchChem are intended solely for informational purposes. The products available for purchase on BenchChem are specifically designed for in-vitro studies, which are conducted outside of living organisms. In-vitro studies, derived from the Latin term "in glass," involve experiments performed in controlled laboratory settings using cells or tissues. It is important to note that these products are not categorized as medicines or drugs, and they have not received approval from the FDA for the prevention, treatment, or cure of any medical condition, ailment, or disease. We must emphasize that any form of bodily introduction of these products into humans or animals is strictly prohibited by law. It is essential to adhere to these guidelines to ensure compliance with legal and ethical standards in research and experimentation.