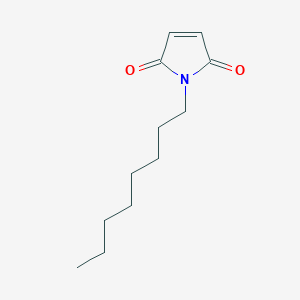
Octyl Maleimide
Overview
Description
N-Octylmaleimide is an organic compound belonging to the maleimide family, characterized by the presence of an octyl group attached to the nitrogen atom of the maleimide ring. This compound is known for its reactivity and versatility in various chemical processes, making it a valuable tool in organic synthesis and industrial applications.
Preparation Methods
Synthetic Routes and Reaction Conditions: N-Octylmaleimide can be synthesized through the reaction of maleic anhydride with octylamine, followed by cyclization to form the maleimide ring. The reaction typically involves heating the reactants in an appropriate solvent, such as toluene, under reflux conditions. The resulting product is then purified through recrystallization or chromatography .
Industrial Production Methods: Industrial production of N-Octylmaleimide follows similar synthetic routes but on a larger scale. The process involves the use of continuous flow reactors to ensure consistent product quality and yield. The reaction conditions are optimized to maximize efficiency and minimize by-products .
Chemical Reactions Analysis
Types of Reactions: N-Octylmaleimide undergoes various chemical reactions, including:
Michael Addition: Reacts with nucleophiles such as thiols and amines to form thioether and amine adducts.
Diels-Alder Reaction: Participates in cycloaddition reactions with dienes to form cyclohexene derivatives.
Substitution Reactions: Undergoes substitution reactions with halogens and other electrophiles
Common Reagents and Conditions:
Michael Addition: Typically conducted in the presence of a base such as triethylamine at room temperature.
Diels-Alder Reaction: Performed under thermal conditions or using a Lewis acid catalyst.
Substitution Reactions: Carried out using halogenating agents like N-bromosuccinimide (NBS) in an inert solvent
Major Products Formed:
Thioether and Amine Adducts: Formed from Michael addition reactions.
Cyclohexene Derivatives: Resulting from Diels-Alder reactions.
Halogenated Maleimides: Produced through substitution reactions
Scientific Research Applications
N-Octylmaleimide has a wide range of applications in scientific research, including:
Mechanism of Action
N-Octylmaleimide exerts its effects through the formation of covalent bonds with nucleophilic groups, such as thiols and amines, in target molecules. This reactivity is primarily due to the electrophilic nature of the maleimide ring, which facilitates nucleophilic attack. The resulting adducts are stable and can modify the biological activity of proteins and other biomolecules .
Comparison with Similar Compounds
N-Ethylmaleimide: Similar in structure but with an ethyl group instead of an octyl group.
N-Phenylmaleimide: Contains a phenyl group and is used in polymer chemistry for the synthesis of high-performance materials.
N-Methylmaleimide: Features a methyl group and is employed in organic synthesis for various cycloaddition reactions.
Uniqueness of N-Octylmaleimide: N-Octylmaleimide is unique due to its longer alkyl chain, which imparts increased hydrophobicity and enhances its ability to interact with lipid membranes and hydrophobic regions of proteins. This property makes it particularly useful in applications requiring membrane permeability and stability .
Biological Activity
Octyl Maleimide (N-Octylmaleimide) is an organic compound belonging to the maleimide family, characterized by its unique structure that includes an octyl group attached to the nitrogen atom of the maleimide ring. This compound has garnered attention due to its diverse biological activities, particularly in antimicrobial, analgesic, and antifungal applications. This article explores the biological activity of this compound, including its mechanisms of action, biochemical interactions, and potential therapeutic uses.
Chemical Structure:
- This compound has a molecular formula of C₁₃H₁₃N₁O₂ and a molecular weight of 219.25 g/mol.
Mechanism of Action:
- The primary mechanism involves a rapid reaction between thiols (from cysteine residues) and maleimides, resulting in the formation of thiosuccinimides. This reaction is crucial for bioconjugation techniques used in modifying proteins and peptides to enhance their stability and functionality.
Antimicrobial Activity
Research indicates that this compound exhibits significant antimicrobial properties. It has been shown to effectively inhibit various fungal pathogens by interfering with the biosynthesis of chitin and β(1,3)glucan, essential components of fungal cell walls. This inhibition leads to compromised cell integrity and eventual cell death .
Analgesic and Antifungal Properties
In addition to its antimicrobial effects, this compound has demonstrated analgesic properties in preclinical studies. Its potential as an antifungal agent is particularly noteworthy against pathogens such as Sclerotinia sclerotiorum and Botrytis cinerea, which are responsible for significant agricultural losses .
Biochemical Interactions
This compound interacts with various enzymes and proteins, influencing cellular functions such as signaling pathways, gene expression, and metabolic processes. The compound's ability to form stable conjugates through Michael addition reactions allows it to be utilized in drug delivery systems and bioimaging applications .
Case Studies
-
Antifungal Efficacy Study:
A study evaluated the efficacy of this compound against Botrytis cinerea in vitro. Results showed a significant reduction in fungal growth at concentrations as low as 100 µg/mL, indicating its potential as a fungicide with lower toxicity compared to traditional agents . -
Bioconjugation Applications:
In a study focused on bioconjugation techniques, this compound was used to modify antibodies for targeted drug delivery. The resulting conjugates exhibited enhanced stability and specificity towards cancer cells, demonstrating promising results in preclinical models .
Pharmacokinetics
The pharmacokinetic profile of this compound suggests that it is relatively stable in physiological conditions, which may enhance its bioavailability. The compound's action is influenced by environmental pH; at neutral pH (7.0), the reaction rate with thiols is significantly accelerated compared to reactions with amines .
Safety and Toxicity
While this compound shows promise in various applications, safety assessments are crucial. Preliminary studies indicate a favorable toxicity profile; however, further research is necessary to comprehensively evaluate its safety for clinical use.
Future Directions
Research into this compound continues to expand, particularly in exploring its potential applications in drug delivery systems and as a therapeutic agent in targeting specific cellular pathways. Ongoing studies aim to elucidate its full range of biological activities and optimize its use in clinical settings.
Q & A
Basic Research Questions
Q. What are the standard synthetic routes for octyl maleimide, and how are purity and structural integrity validated?
- Methodological Answer : this compound is typically synthesized via nucleophilic substitution, where maleic anhydride reacts with octylamine under controlled anhydrous conditions. Structural validation requires (e.g., maleimide proton peaks at δ 6.7–7.0 ppm) and . Purity is assessed using reverse-phase HPLC (C18 column, acetonitrile/water gradient) or LC-MS. For reproducibility, protocols from nanoparticle functionalization studies (e.g., PLGA-PEG maleimide formulations) can be adapted, emphasizing solvent choice (e.g., DMF or THF) to prevent hydrolysis .
Q. What analytical techniques are recommended for quantifying this compound in complex matrices like biological or polymeric systems?
- Methodological Answer : Surface-Enhanced Raman Spectroscopy (SERS) offers semi-quantitative detection (60–120 µg/mL range) with maleimide-specific peaks at 1,660 cm (C=O stretch) and 1,400 cm (C-N-C bend) . For lower detection limits (<10 µg/mL), HPLC-UV (λ = 310 nm) or LC-MS/MS in MRM mode is preferred. Sample preparation should include extraction with organic solvents (e.g., dichloromethane) to isolate this compound from hydrophilic interferents .
Advanced Research Questions
Q. How does the octyl chain influence maleimide’s reactivity and solubility in bioconjugation applications?
- Methodological Answer : The octyl group increases hydrophobicity, reducing aqueous solubility but enhancing compatibility with lipid bilayers or polymeric cores (e.g., PLGA nanoparticles). This can lead to maleimide group burial in hydrophobic regions, as observed in PLGA-PEG nanoparticles, where only 50% of maleimide groups are surface-accessible. To mitigate this, use hydrophilic spacers (e.g., PEG linkers) or characterize accessibility via thiol titration (e.g., Ellman’s assay) .
Q. How can researchers resolve discrepancies between theoretical and experimental ligand conjugation efficiencies in this compound-functionalized nanoparticles?
- Methodological Answer : Discrepancies arise from maleimide burial in nanoparticle cores (e.g., 6 vs. 77 ligands/NP in 10% maleimide formulations). Quantify accessible maleimide via qPAINT (quantitative DNA-PAINT microscopy) or using fluorine-labeled thiols. Cross-reference with cysteine titration assays and adjust formulation parameters (e.g., PEG molecular weight, maleimide density) to optimize surface availability .
Q. What methodological considerations are critical when comparing kinetic data of this compound reactions obtained via UV spectroscopy vs. ?
- Methodological Answer : UV spectroscopy (310 nm) tracks maleimide consumption in real-time but lacks specificity in multicomponent systems. provides direct structural evidence (e.g., maleimide proton disappearance) but requires deuterated solvents and longer acquisition times. Calibrate both methods with internal standards (e.g., maleic acid) and validate activation energy () calculations using Arrhenius plots .
Q. Data Analysis and Contradiction Management
Q. How should researchers address conflicting data on this compound’s stability under physiological conditions?
- Methodological Answer : Stability studies must control pH (maleimide hydrolyzes above pH 7.5) and temperature. Use buffered solutions (e.g., PBS, pH 6.5–7.0) and track hydrolysis via HPLC or loss of thiol reactivity. Conflicting reports often stem from variable buffer compositions or incubation times; replicate conditions from prior studies (e.g., 24-hour stability assays at 37°C) .
Q. What statistical approaches are suitable for analyzing this compound’s dose-response effects in cellular assays?
- Methodological Answer : Use nonlinear regression (e.g., sigmoidal dose-response curves in GraphPad Prism) to calculate EC values. For cytotoxicity assays, apply ANOVA with post-hoc Tukey tests to compare groups, ensuring n ≥ 3 biological replicates. Normalize data to vehicle controls and report confidence intervals to address variability .
Q. Experimental Design and Reproducibility
Q. What protocols ensure reproducibility in this compound-based polymer synthesis?
- Methodological Answer : Document maleimide-to-monomer ratios (e.g., 10–100 mol%), solvent purity (e.g., anhydrous DMF), and reaction times (typically 24–48 hours). For Diels-Alder reactions, specify diene partners (e.g., furan derivatives) and thermal conditions (e.g., 60°C for reversible bonding). Include (glass transition temperature) data via DSC to confirm polymer structure .
Q. How can researchers validate this compound’s role in targeted drug delivery systems?
- Methodological Answer : Use fluorescence quenching assays (e.g., Tide Quencher™ 5WS maleimide with Cy5-labeled ligands) to confirm thiol-specific conjugation. Validate targeting via flow cytometry (e.g., receptor-positive vs. negative cells) and in vivo imaging. Compare release kinetics in reducing (e.g., glutathione-rich) vs. non-reducing environments to confirm disulfide stability .
Properties
IUPAC Name |
1-octylpyrrole-2,5-dione | |
---|---|---|
Source | PubChem | |
URL | https://pubchem.ncbi.nlm.nih.gov | |
Description | Data deposited in or computed by PubChem | |
InChI |
InChI=1S/C12H19NO2/c1-2-3-4-5-6-7-10-13-11(14)8-9-12(13)15/h8-9H,2-7,10H2,1H3 | |
Source | PubChem | |
URL | https://pubchem.ncbi.nlm.nih.gov | |
Description | Data deposited in or computed by PubChem | |
InChI Key |
KIKBJYQCJJXCBZ-UHFFFAOYSA-N | |
Source | PubChem | |
URL | https://pubchem.ncbi.nlm.nih.gov | |
Description | Data deposited in or computed by PubChem | |
Canonical SMILES |
CCCCCCCCN1C(=O)C=CC1=O | |
Source | PubChem | |
URL | https://pubchem.ncbi.nlm.nih.gov | |
Description | Data deposited in or computed by PubChem | |
Molecular Formula |
C12H19NO2 | |
Source | PubChem | |
URL | https://pubchem.ncbi.nlm.nih.gov | |
Description | Data deposited in or computed by PubChem | |
Related CAS |
26714-91-0 | |
Record name | 1H-Pyrrole-2,5-dione, 1-octyl-, homopolymer | |
Source | CAS Common Chemistry | |
URL | https://commonchemistry.cas.org/detail?cas_rn=26714-91-0 | |
Description | CAS Common Chemistry is an open community resource for accessing chemical information. Nearly 500,000 chemical substances from CAS REGISTRY cover areas of community interest, including common and frequently regulated chemicals, and those relevant to high school and undergraduate chemistry classes. This chemical information, curated by our expert scientists, is provided in alignment with our mission as a division of the American Chemical Society. | |
Explanation | The data from CAS Common Chemistry is provided under a CC-BY-NC 4.0 license, unless otherwise stated. | |
DSSTOX Substance ID |
DTXSID60333583 | |
Record name | Octyl Maleimide | |
Source | EPA DSSTox | |
URL | https://comptox.epa.gov/dashboard/DTXSID60333583 | |
Description | DSSTox provides a high quality public chemistry resource for supporting improved predictive toxicology. | |
Molecular Weight |
209.28 g/mol | |
Source | PubChem | |
URL | https://pubchem.ncbi.nlm.nih.gov | |
Description | Data deposited in or computed by PubChem | |
CAS No. |
4080-76-6 | |
Record name | Octyl Maleimide | |
Source | EPA DSSTox | |
URL | https://comptox.epa.gov/dashboard/DTXSID60333583 | |
Description | DSSTox provides a high quality public chemistry resource for supporting improved predictive toxicology. | |
Synthesis routes and methods
Procedure details
Retrosynthesis Analysis
AI-Powered Synthesis Planning: Our tool employs the Template_relevance Pistachio, Template_relevance Bkms_metabolic, Template_relevance Pistachio_ringbreaker, Template_relevance Reaxys, Template_relevance Reaxys_biocatalysis model, leveraging a vast database of chemical reactions to predict feasible synthetic routes.
One-Step Synthesis Focus: Specifically designed for one-step synthesis, it provides concise and direct routes for your target compounds, streamlining the synthesis process.
Accurate Predictions: Utilizing the extensive PISTACHIO, BKMS_METABOLIC, PISTACHIO_RINGBREAKER, REAXYS, REAXYS_BIOCATALYSIS database, our tool offers high-accuracy predictions, reflecting the latest in chemical research and data.
Strategy Settings
Precursor scoring | Relevance Heuristic |
---|---|
Min. plausibility | 0.01 |
Model | Template_relevance |
Template Set | Pistachio/Bkms_metabolic/Pistachio_ringbreaker/Reaxys/Reaxys_biocatalysis |
Top-N result to add to graph | 6 |
Feasible Synthetic Routes
Disclaimer and Information on In-Vitro Research Products
Please be aware that all articles and product information presented on BenchChem are intended solely for informational purposes. The products available for purchase on BenchChem are specifically designed for in-vitro studies, which are conducted outside of living organisms. In-vitro studies, derived from the Latin term "in glass," involve experiments performed in controlled laboratory settings using cells or tissues. It is important to note that these products are not categorized as medicines or drugs, and they have not received approval from the FDA for the prevention, treatment, or cure of any medical condition, ailment, or disease. We must emphasize that any form of bodily introduction of these products into humans or animals is strictly prohibited by law. It is essential to adhere to these guidelines to ensure compliance with legal and ethical standards in research and experimentation.