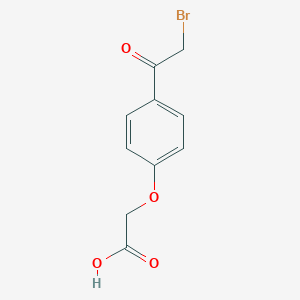
PTP Inhibitor III
Overview
Description
PTP Inhibitor III is a cell-permeable compound that inhibits a broad range of protein tyrosine phosphatases. Protein tyrosine phosphatases are enzymes that remove phosphate groups from phosphorylated tyrosine residues on proteins, playing a crucial role in regulating cellular signaling pathways. This compound is particularly significant in research due to its ability to modulate these pathways, making it a valuable tool in the study of various diseases, including cancer and diabetes .
Mechanism of Action
Target of Action
PTP Inhibitor III, also known as 2-[4-(2-bromoacetyl)phenoxy]acetic Acid or 4-(bromoacetyl)phenoxyacetic acid, primarily targets Protein Tyrosine Phosphatases (PTPs) . PTPs are enzymes that remove phosphate groups from phosphorylated tyrosine residues on proteins . They play a crucial role in cellular signaling and are involved in various physiological processes .
Mode of Action
This compound acts as an inhibitor of PTPs . It binds to the active site of the PTPs, thereby preventing them from dephosphorylating their substrate proteins . This inhibition can alter the phosphorylation state of various proteins, affecting the signaling pathways they are involved in .
Biochemical Pathways
The inhibition of PTPs by this compound affects several biochemical pathways. PTPs are involved in numerous signaling pathways, including those related to cell growth, differentiation, and metabolism . By inhibiting PTPs, this compound can potentially affect these pathways, leading to changes in cellular functions .
Pharmacokinetics
The effectiveness of any drug is influenced by its pharmacokinetic properties, which determine the drug’s bioavailability, or the extent and rate at which the active moiety (drug or metabolite) enters systemic circulation, thereby accessing the site of action .
Result of Action
The inhibition of PTPs by this compound can lead to various molecular and cellular effects. For instance, it can alter the phosphorylation state of proteins, affecting their activity and the cellular functions they regulate . This can have potential therapeutic implications in diseases where PTPs are known to play a role, such as cancer, diabetes, and autoimmune disorders .
Action Environment
The action, efficacy, and stability of this compound can be influenced by various environmental factors. These can include the presence of other molecules, pH, temperature, and the specific cellular environment . .
Biochemical Analysis
Biochemical Properties
PTP Inhibitor III plays a significant role in biochemical reactions. It binds the catalytic domain of SHP-1 and covalently reacts with free thiols . This reaction is reversible with irradiation . It inhibits a broad range of PTPs , which are enzymes that remove phosphate from a substrate, resulting in a phosphate ion and a hydroxyl group on the substrate .
Cellular Effects
This compound has profound effects on various types of cells and cellular processes. It influences cell function by balancing the activation of signaling proteins in vascular cells . This helps keep the cells functioning in a normal state .
Molecular Mechanism
At the molecular level, this compound exerts its effects through binding interactions with biomolecules and enzyme inhibition. It binds covalently to the active site of the enzyme . This binding interaction is crucial for its inhibitory action on PTPs .
Metabolic Pathways
This compound is involved in metabolic pathways that involve PTPs
Preparation Methods
Synthetic Routes and Reaction Conditions: PTP Inhibitor III, formally known as 2-[4-(2-bromoacetyl)phenoxy]-acetic acid, is synthesized through a series of chemical reactionsThe reaction conditions often require the use of solvents like dimethylformamide or dimethyl sulfoxide and catalysts to facilitate the reactions .
Industrial Production Methods: While specific industrial production methods for this compound are not extensively documented, the general approach involves scaling up the laboratory synthesis process. This includes optimizing reaction conditions to ensure high yield and purity, as well as implementing safety measures to handle the reactive intermediates and final product.
Chemical Reactions Analysis
Types of Reactions: PTP Inhibitor III undergoes several types of chemical reactions, including:
Oxidation: The compound can be oxidized under specific conditions, altering its chemical structure and potentially its inhibitory activity.
Reduction: Reduction reactions can modify the bromine atom, affecting the compound’s reactivity.
Common Reagents and Conditions:
Oxidation: Common oxidizing agents include hydrogen peroxide and potassium permanganate.
Reduction: Reducing agents such as sodium borohydride or lithium aluminum hydride are often used.
Substitution: Nucleophilic reagents like amines or thiols can be used to substitute the bromine atom.
Major Products Formed: The major products formed from these reactions depend on the specific reagents and conditions used. For example, substitution reactions can yield a variety of derivatives with different functional groups replacing the bromine atom.
Scientific Research Applications
PTP Inhibitor III has a wide range of scientific research applications, including:
Chemistry: Used as a tool to study the inhibition of protein tyrosine phosphatases and their role in cellular signaling pathways.
Biology: Helps in understanding the regulation of cellular processes such as cell growth, differentiation, and apoptosis.
Medicine: Investigated for its potential therapeutic applications in treating diseases like cancer, diabetes, and autoimmune disorders by modulating protein tyrosine phosphatase activity
Industry: Utilized in the development of new drugs and therapeutic agents targeting protein tyrosine phosphatases.
Comparison with Similar Compounds
Vanadium Compounds: These compounds also inhibit protein tyrosine phosphatases but through different mechanisms, often involving the mimicry of phosphate groups.
Bisperoxo (1,10-phenanthroline)oxidovanadate (V): Another inhibitor that targets protein tyrosine phosphatases, but with a distinct chemical structure and mode of action.
Bis (maltolato)oxidovanadium (IV): Known for its potential in treating diabetes and cancer by inhibiting protein tyrosine phosphatases.
Uniqueness of PTP Inhibitor III: this compound is unique due to its photoreversible covalent inhibition mechanism, allowing for precise control over enzyme activity. This feature makes it particularly valuable in research settings where temporal control of enzyme inhibition is crucial.
Properties
IUPAC Name |
2-[4-(2-bromoacetyl)phenoxy]acetic acid | |
---|---|---|
Source | PubChem | |
URL | https://pubchem.ncbi.nlm.nih.gov | |
Description | Data deposited in or computed by PubChem | |
InChI |
InChI=1S/C10H9BrO4/c11-5-9(12)7-1-3-8(4-2-7)15-6-10(13)14/h1-4H,5-6H2,(H,13,14) | |
Source | PubChem | |
URL | https://pubchem.ncbi.nlm.nih.gov | |
Description | Data deposited in or computed by PubChem | |
InChI Key |
RLSUZPAAQRBGTM-UHFFFAOYSA-N | |
Source | PubChem | |
URL | https://pubchem.ncbi.nlm.nih.gov | |
Description | Data deposited in or computed by PubChem | |
Canonical SMILES |
C1=CC(=CC=C1C(=O)CBr)OCC(=O)O | |
Source | PubChem | |
URL | https://pubchem.ncbi.nlm.nih.gov | |
Description | Data deposited in or computed by PubChem | |
Molecular Formula |
C10H9BrO4 | |
Source | PubChem | |
URL | https://pubchem.ncbi.nlm.nih.gov | |
Description | Data deposited in or computed by PubChem | |
Molecular Weight |
273.08 g/mol | |
Source | PubChem | |
URL | https://pubchem.ncbi.nlm.nih.gov | |
Description | Data deposited in or computed by PubChem | |
Synthesis routes and methods
Procedure details
Retrosynthesis Analysis
AI-Powered Synthesis Planning: Our tool employs the Template_relevance Pistachio, Template_relevance Bkms_metabolic, Template_relevance Pistachio_ringbreaker, Template_relevance Reaxys, Template_relevance Reaxys_biocatalysis model, leveraging a vast database of chemical reactions to predict feasible synthetic routes.
One-Step Synthesis Focus: Specifically designed for one-step synthesis, it provides concise and direct routes for your target compounds, streamlining the synthesis process.
Accurate Predictions: Utilizing the extensive PISTACHIO, BKMS_METABOLIC, PISTACHIO_RINGBREAKER, REAXYS, REAXYS_BIOCATALYSIS database, our tool offers high-accuracy predictions, reflecting the latest in chemical research and data.
Strategy Settings
Precursor scoring | Relevance Heuristic |
---|---|
Min. plausibility | 0.01 |
Model | Template_relevance |
Template Set | Pistachio/Bkms_metabolic/Pistachio_ringbreaker/Reaxys/Reaxys_biocatalysis |
Top-N result to add to graph | 6 |
Feasible Synthetic Routes
Q1: How does PTP Inhibitor III modulate TRPV1 activity in the context of inflammatory pain?
A: this compound is a non-selective inhibitor of protein tyrosine phosphatases (PTPs). [] Research suggests that it indirectly enhances the activity of TRPV1, a receptor heavily implicated in pain signaling. The mechanism involves inhibiting the activity of SHP-1, a specific PTP that normally dephosphorylates and downregulates TRPV1. By inhibiting SHP-1, this compound allows for increased tyrosine phosphorylation of TRPV1, leading to its sensitization and amplified pain signaling. [] This effect was observed both in vitro, using cultured DRG neurons, and in vivo, where intrathecal administration of this compound induced thermal hyperalgesia in rats. [] Importantly, this hyperalgesia was effectively blocked by pre-treatment with TRPV1 antagonists, confirming the involvement of TRPV1 in this pathway. []
Q2: What is the significance of studying SHP-1 and its interplay with this compound in inflammatory pain models?
A: Research indicates that SHP-1 plays a crucial role in regulating TRPV1 activity and, consequently, pain sensation. [] In the provided study, CFA-induced inflammatory pain in rats led to an increase in SHP-1 expression in DRG neurons, alongside increased TRPV1 expression and phosphorylation. [] This suggests a compensatory mechanism where SHP-1 upregulation attempts to dampen the increased TRPV1 activity and alleviate excessive pain. Further supporting this, overexpressing SHP-1 in DRG neurons actually reduced CFA-induced inflammatory pain in the rat model. [] Therefore, studying the interactions between SHP-1 and this compound can provide valuable insights into the complex mechanisms of inflammatory pain and potentially identify novel therapeutic targets.
Disclaimer and Information on In-Vitro Research Products
Please be aware that all articles and product information presented on BenchChem are intended solely for informational purposes. The products available for purchase on BenchChem are specifically designed for in-vitro studies, which are conducted outside of living organisms. In-vitro studies, derived from the Latin term "in glass," involve experiments performed in controlled laboratory settings using cells or tissues. It is important to note that these products are not categorized as medicines or drugs, and they have not received approval from the FDA for the prevention, treatment, or cure of any medical condition, ailment, or disease. We must emphasize that any form of bodily introduction of these products into humans or animals is strictly prohibited by law. It is essential to adhere to these guidelines to ensure compliance with legal and ethical standards in research and experimentation.