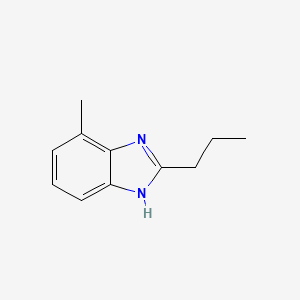
4-Methyl-2-propyl-1H-benzimidazole
Overview
Description
4-Methyl-2-propyl-1H-benzimidazole (C₁₁H₁₄N₂) is a heterocyclic aromatic compound featuring a benzimidazole core substituted with a methyl group at the 4-position and a propyl group at the 2-position. Benzimidazoles are known for their diverse biological activities, including antimicrobial, antiviral, and anticancer properties, often attributed to their ability to interact with biomolecules like DNA or enzymes . The substitution pattern in this compound may influence its electronic properties, lipophilicity, and binding affinity, making it a subject of interest in medicinal chemistry and materials science.
Scientific Research Applications
Chemical Synthesis and Derivatives
MPB serves as a critical intermediate in the synthesis of various bioactive compounds, most notably angiotensin II receptor blockers (ARBs) like telmisartan. The synthesis of MPB involves several methods, including:
- Amidation Reactions : Utilizing N,N'-carbonyldiimidazole to activate carboxylic acids for amidation, leading to high yields and purity of the target compound .
- Reflux Techniques : The compound can be synthesized through refluxing processes involving various solvents and reagents, optimizing reaction conditions to enhance yield and minimize byproducts .
Angiotensin II Receptor Blockade
MPB is integral in the development of ARBs. Telmisartan, derived from MPB, is used for managing hypertension and heart failure. The mechanism involves blocking the angiotensin II type 1 receptor (AT1R), which leads to vasodilation and reduced blood pressure .
PPARγ Activation
Research indicates that MPB derivatives exhibit peroxisome proliferator-activated receptor gamma (PPARγ) agonist activity. PPARγ plays a crucial role in glucose metabolism and fat cell differentiation, making these compounds potential candidates for diabetes management .
Industrial Applications
The industrial synthesis of MPB has been optimized for scalability. The methods developed allow for:
- High Yield Production : New synthetic routes have been established that provide high yields while being cost-effective and environmentally friendly .
- Pharmaceutical Manufacturing : MPB's derivatives are vital in producing various medications, enhancing the pharmaceutical industry's ability to meet market demands efficiently.
Telmisartan Synthesis
A notable case study involves the synthesis of telmisartan from MPB. The process highlights:
- Yield Optimization : Using refined methods, researchers achieved over 85% yield in producing telmisartan from its precursors, demonstrating the efficiency of MPB in pharmaceutical applications .
- Purity Assessment : The resultant telmisartan exhibited high purity levels suitable for clinical use, underscoring the importance of MPB as a precursor .
PPARγ Agonists Development
Another study focused on developing new PPARγ agonists based on MPB derivatives:
- Biological Evaluation : These derivatives were tested for their ability to activate PPARγ, showing promising results that could lead to new therapeutic agents for metabolic disorders .
Comparative Data Table
Application | Compound Derived | Yield (%) | Biological Activity |
---|---|---|---|
Angiotensin II Blocker | Telmisartan | 85 | Hypertension management |
PPARγ Agonist | Various MPB Derivatives | >70 | Diabetes treatment potential |
Chemical Reactions Analysis
Cyclization Reactions
The core benzimidazole structure is synthesized via cyclization of nitro-precursors under reductive conditions. A prominent method involves:
Condensation Reactions
The compound serves as a precursor for synthesizing bis-benzimidazole derivatives, which are pharmacologically relevant.
Comparative Analysis of Synthetic Routes
The choice of solvent and catalyst significantly impacts efficiency and safety:
Method | Catalyst/Solvent | Temperature | Yield | Advantages |
---|---|---|---|---|
Polyphosphoric acid | Nonpolar solvent | 130–135°C | 77.4% | High purity, operator-safe |
Phosphoric acid | Polar solvent | 150–155°C | 60.5% | Lower cost, scalable |
P₂O₅/MSA | Methanesulfonic acid | 125–145°C | 85% | High yield but hazardous |
The polyphosphoric acid route is industrially preferred due to safety and reduced reagent excess .
Oxidation and Reduction
-
Oxidation : The benzimidazole ring undergoes oxidation with agents like hydrogen peroxide, forming hydroxylated derivatives.
-
Reduction : Sodium borohydride reduces nitro intermediates to amines during cyclization .
Substitution Reactions
-
Nucleophilic substitution at the C-6 position is feasible using halogens or alkylating agents, enabling further derivatization .
Stability and Reaction Optimization
-
Temperature Sensitivity : Degradation occurs above 155°C, necessitating precise thermal control .
-
pH Dependence : Cyclization requires alkaline conditions (pH 10–11) to stabilize intermediates .
Key Industrial Considerations
Q & A
Basic Research Questions
Q. What are the optimal synthetic conditions for achieving high-purity 4-Methyl-2-propyl-1H-benzimidazole?
- Methodological Answer : High purity (≥95%) can be achieved via refluxing intermediates with potassium carbonate (K₂CO₃) and sodium methoxide in methanol, followed by extraction with ethyl acetate and crystallization at 0°C. Purity should be validated using HPLC, as demonstrated in the synthesis of analogous compounds .
Q. Which spectroscopic techniques are most reliable for characterizing substituent effects on the benzimidazole core?
- Methodological Answer : Infrared (IR) spectroscopy identifies functional groups (e.g., N-H stretches at ~3400 cm⁻¹), while ¹H/¹³C NMR confirms substituent positions (e.g., propyl chain integration at δ ~0.9–1.6 ppm). Mass spectrometry (ESI-MS) provides molecular weight validation, as shown for pyridyl-substituted benzimidazoles .
Q. How can researchers ensure reproducibility in synthesizing benzimidazole derivatives under mild conditions?
- Methodological Answer : Use catalytic tetra-n-butylammonium bromide with alkyl halides, as demonstrated in the alkylation of benzimidazol-2-one derivatives. Reaction progress should be monitored via TLC, and products purified via column chromatography .
Advanced Research Questions
Q. How can crystallographic data discrepancies in this compound derivatives be resolved?
- Methodological Answer : Refine structural models using SHELXL, which is robust for small-molecule crystallography. Cross-validate hydrogen bonding and π-π interactions with spectroscopic data (e.g., NMR coupling constants). For example, the monoclinic P2₁/c space group was confirmed for a related hydroxy-methoxybenzyl derivative via SHELX refinement .
Q. What strategies address contradictions in biological activity data among structurally similar benzimidazole analogs?
- Methodological Answer : Perform comparative structure-activity relationship (SAR) studies by systematically varying substituents (e.g., aryl thiazole-triazole groups) and correlating with activity assays. Molecular docking (e.g., AutoDock Vina) can predict binding poses, as seen in analogs targeting enzyme active sites .
Q. How can hydrogen bonding networks in this compound crystals be analyzed quantitatively?
- Methodological Answer : Use Mercury Software to calculate hydrogen bond distances and angles from Crystallographic Information Files (CIFs). For example, the title compound’s crystal structure revealed O-H···N interactions with bond lengths of 1.82–1.89 Å .
Q. What computational methods are suitable for predicting the stability of benzimidazole derivatives under thermal stress?
- Methodological Answer : Perform thermogravimetric analysis (TGA) coupled with density functional theory (DFT) to model decomposition pathways. For instance, a pyridyl-benzimidazole derivative exhibited 10% mass loss at 220°C, aligning with DFT-predicted bond dissociation energies .
Q. Data Analysis and Contradiction Management
Q. How should researchers handle conflicting solubility data for this compound in polar vs. non-polar solvents?
- Methodological Answer : Re-evaluate purity via HPLC and control for hydration states. For example, ethyl acetate/water partitioning in analogous compounds improved solubility consistency by removing hydrophilic impurities .
Q. What experimental controls are critical when comparing catalytic efficiencies in benzimidazole functionalization?
Comparison with Similar Compounds
Comparison with Structurally Similar Compounds
Structural and Functional Differences
The following table summarizes key structural and physicochemical properties of 4-Methyl-2-propyl-1H-benzimidazole and its analogs:
Key Findings from Comparative Analysis
Substitution Position and Electronic Effects: The propyl group in the 2-position of the target compound contrasts with the 1-position propyl in 2-(4-Methoxyphenyl)-1-propyl-1H-benzimidazole . The methoxy group in the analog from enhances electron-donating capacity, which could increase solubility but reduce metabolic stability compared to the methyl group in the target compound.
The sulfanyl-phenoxypropyl chain in ’s compound introduces sulfur-based electronic effects and extended hydrophobicity, which might influence redox activity or solubility in nonpolar environments .
Aromatic Bulk and Bioavailability :
- Compounds like 1-(4-Methylbenzyl)-2-(4-methylphenyl)-1H-benzimidazole feature bulky aromatic substituents, which may enhance target specificity but reduce bioavailability due to increased steric hindrance and logP values.
Preparation Methods
Preparation Methods
Synthesis via Activation and Amidation of 4-Methyl-2-propyl-1H-benzimidazole-6-carboxylic Acid
One effective preparation method involves the activation of this compound-6-carboxylic acid using N,N'-carbonyldiimidazole (CDI), followed by amidation to produce carboxamide derivatives. The process addresses common issues such as poor solubility, low yield, and environmental concerns associated with traditional methods.
Step | Description | Conditions |
---|---|---|
1 | Dissolve this compound-6-carboxylic acid in a suitable solvent. | Room temperature, stirring >30 min |
2 | Add N,N'-carbonyldiimidazole to activate the acid group. | Room temperature |
3 | Add amine compound to the activated mixture. | 20–100°C, stir for 2–24 hours |
4 | Remove solvent under reduced pressure, add water to precipitate product, filter and dry. | Ambient temperature |
This method yields high purity products with improved reaction efficiency and reduced environmental impact.
Reduction of Nitro Precursors to Obtain this compound-6-carboxylic Acid
A key intermediate in the synthesis of this compound is the 6-carboxylic acid derivative. This can be prepared by reducing methyl 4-(butyrylamino)-3-methyl-5-nitrobenzoate using sodium dithionite in aqueous media.
Step | Description | Conditions | Yield |
---|---|---|---|
1 | Suspend methyl 4-(butyrylamino)-3-methyl-5-nitrobenzoate in water and heat to 70–80°C. | 70–80°C | |
2 | Gradually add sodium dithionite suspension, raise temperature to 90–100°C. | 90–100°C | |
3 | Adjust pH to 10–11, stir until starting material disappears (monitored by TLC). | pH 10–11, 90–100°C | |
4 | Adjust pH to 6–6.5, filter and dry solid product. | Ambient temperature | 96.4% |
This reduction step is efficient and provides a high yield of the carboxylic acid intermediate critical for further transformations.
Cyclization in Polyphosphoric Acid for Benzimidazole Core Formation
Another approach involves cyclizing 2-n-propyl-4-methyl-1H-benzimidazole-6-carboxylic acid with N-methyl-o-phenylenediamine in polyphosphoric acid (PPA) or phosphoric acid to form substituted benzimidazole derivatives.
Step | Description | Conditions | Yield |
---|---|---|---|
1 | Suspend 2-n-propyl-4-methyl-1H-benzimidazole-6-carboxylic acid and N-methyl-o-phenylenediamine in PPA or phosphoric acid. | 70–75°C to 130–135°C, 10 hours | 60.5–85% |
2 | Cool reaction mixture, neutralize with ammonia or sodium hydroxide solution. | 50–70°C | |
3 | Filter solid product and dry. | Ambient temperature |
This method is scalable for industrial production and yields the target benzimidazole derivatives with good efficiency.
Comparative Data Table of Preparation Methods
Method | Starting Material(s) | Key Reagents/Conditions | Yield (%) | Advantages | Disadvantages |
---|---|---|---|---|---|
CDI Activation and Amidation | This compound-6-carboxylic acid | N,N'-Carbonyldiimidazole, amines, 20–100°C | High (not specified) | High purity, environmentally friendly | Requires pure acid intermediate |
Sodium Dithionite Reduction | Methyl 4-(butyrylamino)-3-methyl-5-nitrobenzoate | Sodium dithionite, water, 70–100°C | 96.4 | High yield, simple reagents | Requires nitro precursor synthesis |
Cyclization in Polyphosphoric Acid | 2-n-Propyl-4-methyl-1H-benzimidazole-6-carboxylic acid + N-methyl-o-phenylenediamine | PPA or phosphoric acid, 70–135°C, 10 hours | 60.5–85 | Suitable for large scale | High temperature, corrosive reagents |
Research Findings and Analysis
- The CDI activation method improves solubility and reaction yield compared to direct amidation techniques, reducing environmental waste by avoiding harsh reagents.
- Sodium dithionite reduction is a mild and efficient method for converting nitrobenzoate precursors to the corresponding benzimidazole carboxylic acids, essential for downstream synthesis.
- Cyclization in polyphosphoric acid is a classical and robust method to form benzimidazole rings, widely used in industrial settings despite requiring high temperatures and corrosive acids.
- Optimization of reaction parameters such as temperature, pH, and reagent stoichiometry significantly affects yield and purity, as demonstrated in patent literature and synthetic studies.
Properties
CAS No. |
99840-45-6 |
---|---|
Molecular Formula |
C11H14N2 |
Molecular Weight |
174.24 g/mol |
IUPAC Name |
4-methyl-2-propyl-1H-benzimidazole |
InChI |
InChI=1S/C11H14N2/c1-3-5-10-12-9-7-4-6-8(2)11(9)13-10/h4,6-7H,3,5H2,1-2H3,(H,12,13) |
InChI Key |
KKECAJNLCGRKJL-UHFFFAOYSA-N |
SMILES |
CCCC1=NC2=C(C=CC=C2N1)C |
Canonical SMILES |
CCCC1=NC2=C(C=CC=C2N1)C |
Origin of Product |
United States |
Synthesis routes and methods
Procedure details
Disclaimer and Information on In-Vitro Research Products
Please be aware that all articles and product information presented on BenchChem are intended solely for informational purposes. The products available for purchase on BenchChem are specifically designed for in-vitro studies, which are conducted outside of living organisms. In-vitro studies, derived from the Latin term "in glass," involve experiments performed in controlled laboratory settings using cells or tissues. It is important to note that these products are not categorized as medicines or drugs, and they have not received approval from the FDA for the prevention, treatment, or cure of any medical condition, ailment, or disease. We must emphasize that any form of bodily introduction of these products into humans or animals is strictly prohibited by law. It is essential to adhere to these guidelines to ensure compliance with legal and ethical standards in research and experimentation.