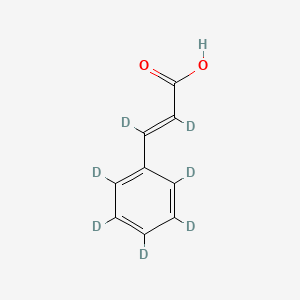
trans-Cinnamic-d7 acid
Overview
Description
trans-Cinnamic acid (3-phenyl-2-propenoic acid) is a naturally occurring phenolic compound belonging to the phenylpropanoid family. It is characterized by a benzene ring attached to an unsaturated carboxylic acid chain. This compound is widely distributed in plants, such as Cinnamomum species, Scutellaria baicalensis, and Amaranthus tricolor, where it functions as a secondary metabolite involved in stress responses and defense mechanisms . trans-Cinnamic acid is also a precursor for biosynthesis of more complex phenylpropanoids, including lignin, coumarins, and flavonoids .
trans-Cinnamic-d7 acid is a deuterated analog of trans-cinnamic acid, where seven hydrogen atoms are replaced with deuterium.
Preparation Methods
Synthetic Routes and Reaction Conditions
Catalytic Deuteration of trans-Cinnamic Acid
- Method : The most common laboratory synthesis involves catalytic hydrogenation of trans-Cinnamic acid in the presence of deuterium gas (D₂). This reaction replaces the vinyl hydrogens with deuterium atoms, yielding trans-Cinnamic-d7 acid.
- Catalysts : Common catalysts include palladium on carbon (Pd/C), platinum, or other noble metals capable of facilitating hydrogen-deuterium exchange.
- Reaction Conditions :
- Temperature: Typically ambient to moderate temperatures (25–80°C).
- Pressure: Deuterium gas pressure ranges from atmospheric to several atmospheres to drive the reaction.
- Solvent: Often carried out in organic solvents such as ethanol, methanol, or ethyl acetate.
- Outcome : High deuterium incorporation (>95%) at the vinyl positions is achievable with optimized conditions.
Industrial Scale Preparation
- Scale-up Considerations : Industrial production follows similar principles but employs specialized equipment to handle deuterium gas safely and efficiently.
- Purification : Advanced purification techniques, such as recrystallization and chromatography, are used to ensure high purity and isotopic enrichment.
- Yield and Quality Control : Emphasis on maximizing yield and maintaining isotopic purity; analytical methods such as NMR and mass spectrometry confirm deuterium incorporation.
Alternative Synthetic Method: Knoevenagel-Doebner Reaction in Ionic Liquids
While the above methods focus on deuteration of existing trans-Cinnamic acid, alternative synthetic routes for trans-Cinnamic acid derivatives (which could be adapted for deuterated analogs) include the Knoevenagel-Doebner condensation of aromatic aldehydes with malonic acid.
- Innovative Solvent System : The use of water-insoluble ionic liquid 1-butyl-3-methylimidazolium hexafluorophosphate ([bmim]PF₆) as a green solvent reduces environmental impact compared to traditional pyridine solvents.
- Catalyst : Piperidine is used catalytically.
- Reaction Conditions :
- Temperature: 80–90°C
- Reaction Time: 4–8 hours
- Advantages : High yield, easy product separation by alkaline aqueous extraction, and recyclability of ionic liquid solvent.
- Potential for Deuterated Compounds : By using deuterated aromatic aldehydes or malonic acid, this method could be adapted for the synthesis of this compound derivatives.
Summary Table of Preparation Methods
Preparation Method | Key Reagents/Conditions | Advantages | Notes |
---|---|---|---|
Catalytic Deuteration | trans-Cinnamic acid, D₂ gas, Pd/C catalyst | High isotopic purity, straightforward | Standard method for this compound |
Reduction with Sodium Borodeuteride | trans-Cinnamic acid, NaBD₄ | Mild conditions, selective deuteration | Produces related hydrocinnamic acid-d7 |
Knoevenagel-Doebner in Ionic Liquid | Aromatic aldehyde, malonic acid, piperidine, [bmim]PF₆ | Green solvent, recyclable, high yield | Adaptable for deuterated precursors |
Research Findings and Analytical Data
- Isotopic Incorporation : Studies confirm that catalytic hydrogenation in deuterium gas achieves >95% deuterium incorporation at the vinyl positions of trans-Cinnamic acid, verified by ^2H NMR and mass spectrometry.
- Kinetic Isotope Effect (KIE) : Deuterium substitution at the β-position slows reaction rates in oxidation and reduction reactions due to KIE, affecting reaction kinetics and metabolic studies.
- Purity and Yield : Industrial processes achieve high yields (typically >85%) with purity suitable for biochemical and pharmacological applications.
Chemical Reactions Analysis
Oxidation Reactions
trans-Cinnamic-d7 acid undergoes oxidation at the α,β-unsaturated double bond. Common oxidizing agents like potassium permanganate (KMnO₄) or chromium trioxide (CrO₃) convert the compound into deuterated cinnamic aldehyde or ketone derivatives. For example:
Deuterium labeling at the β-position slows reaction rates due to the kinetic isotope effect (KIE), though specific quantitative data for this compound remain sparse in published literature .
Reduction Reactions
The double bond in this compound is susceptible to catalytic hydrogenation. Using deuterium gas (D₂) or deuterated reducing agents like sodium borodeuteride (NaBD₄), the compound is reduced to hydrocinnamic acid-d₇:
Deuterium incorporation enhances isotopic tracing in metabolic studies, as observed in biosynthetic pathways involving phenylalanine ammonia-lyase (PAL) .
Electrophilic Aromatic Substitution
The benzene ring undergoes electrophilic substitution, with bromination and nitration being well-documented:
Reaction | Reagents/Conditions | Major Product |
---|---|---|
Bromination | Br₂, FeBr₃ | 4-Bromo-trans-cinnamic-d₇ acid |
Nitration | HNO₃, H₂SO₄ | 3-Nitro-trans-cinnamic-d₇ acid |
Deuterium at the ortho and para positions influences regioselectivity, with meta products favored due to steric and electronic effects .
Esterification and Amidation
The carboxylic acid group participates in esterification and amidation, forming deuterated derivatives with industrial and pharmacological applications:
Key Examples:
-
Esterification : Reaction with methanol (CD₃OD) under acidic conditions yields methyl trans-cinnamate-d₇, a flavoring agent .
-
Amidation : Conjugation with glycyrrhetinic acid via amide bonds produces antitumor agents (e.g., compound 45c in studies), demonstrating enhanced cytotoxicity against cancer cell lines .
Biochemical Reactions
In plant metabolism, this compound serves as a precursor for phenolic compounds. Enzymatic hydroxylation by cytochrome P450 monooxygenases introduces hydroxyl groups, forming deuterated coumaric acid derivatives. Notably, feeding experiments in cassava roots revealed deuterium loss at the C-2 position during scopoletin biosynthesis, suggesting enzymatic H/D exchange mechanisms :
Isotopic Exchange and Stability
Deuterium labels at the α and β positions exhibit stability under standard conditions but undergo exchange in strongly acidic or basic environments. For instance, prolonged exposure to D₂O at pH >10 results in partial H/D exchange at the α-position, as confirmed by mass spectrometry .
Scientific Research Applications
Chemistry
- Tracer in Metabolic Studies : trans-Cinnamic-d7 acid serves as a tracer to study metabolic pathways. Its deuterium labeling allows researchers to trace the compound through various chemical processes, providing insights into reaction mechanisms and kinetics.
Biology
- Enzyme Kinetics : In biological research, this compound is utilized to study enzyme kinetics and metabolic pathways. The stable isotope labeling helps elucidate the metabolic fate of cinnamic acid in biological systems.
- Antimicrobial Activity : The compound exhibits antimicrobial properties, particularly against the fish pathogen Aeromonas sobria, with a minimal inhibitory concentration (MIC) of 250 μg/mL . This application is significant for developing treatments against bacterial infections in aquaculture.
Medicine
- Pharmacokinetic Studies : In pharmacology, this compound is used to evaluate the absorption, distribution, metabolism, and excretion (ADME) of drugs. Deuterium labeling helps distinguish the compound from non-labeled counterparts in complex biological matrices, enhancing the accuracy of pharmacokinetic assessments.
- Cancer Research : Cinnamic acid derivatives have been explored for their potential in treating various cancers. For instance, compounds derived from cinnamic acid have shown cytotoxic effects against cancer cell lines such as MCF-7 (breast cancer) and HeLa (cervical cancer) .
Industry
- Material Development : In industrial applications, this compound is valuable for developing new materials and chemical processes. Its unique properties facilitate the synthesis of high-value chemicals and pharmaceuticals.
Case Study 1: Antimicrobial Efficacy
A study investigated the antimicrobial activity of this compound against Aeromonas sobria. The results indicated that at a concentration of 250 μg/mL, the compound effectively inhibited bacterial growth, suggesting its potential use as an antimicrobial agent in aquaculture settings.
Compound | MIC (μg/mL) | Target Pathogen |
---|---|---|
This compound | 250 | Aeromonas sobria |
Case Study 2: Pharmacokinetics in Drug Development
In pharmacokinetic studies involving deuterated compounds, researchers utilized this compound to assess drug metabolism. The incorporation of deuterium allowed for clearer differentiation between labeled and unlabeled compounds during analysis, leading to improved understanding of drug behavior in biological systems.
Mechanism of Action
The mechanism of action of trans-Cinnamic-d7 acid involves its interaction with various molecular targets and pathways. The compound exerts its effects through the following mechanisms:
Enzyme Inhibition: this compound can inhibit specific enzymes involved in metabolic pathways, leading to altered metabolic flux.
Radical Scavenging: The compound exhibits antioxidant properties by scavenging free radicals and preventing oxidative damage.
Signal Modulation: this compound can modulate signaling pathways, influencing cellular responses and gene expression.
Comparison with Similar Compounds
trans-Cinnamic acid shares structural and functional similarities with other hydroxycinnamic acids and derivatives. Below is a detailed comparison based on structural features, biological activities, and applications.
Structural and Functional Differences
Table 1: Structural Comparison of trans-Cinnamic Acid and Key Analogs
Bioactivity and Efficacy
Table 2: Bioactivity Comparison
Key Research Findings
Geroscience Applications :
- trans-Cinnamic acid at 50 µmol/L increased C. elegans lifespan by 9.8% and improved oxidative stress resistance, though it reduced thermotolerance . Comparatively, caffeic acid and chlorogenic acid exhibit stronger antioxidant effects but lack direct geroprotective evidence in this model .
Para-hydroxylation (p-coumaric acid) improves radical scavenging but reduces antifungal potency relative to caffeic acid .
Natural Abundance :
- trans-Cinnamic acid content varies widely across species:
Biological Activity
Introduction
trans-Cinnamic-d7 acid, a deuterated form of trans-cinnamic acid, is increasingly recognized for its biological activity, particularly in antimicrobial and biochemical applications. This article delves into its mechanism of action, biochemical properties, and various research findings that highlight its significance in both biological and industrial contexts.
Target of Action
The primary target of this compound is the pathogenic bacterium Aeromonas sobria, which poses a significant threat to aquatic life. Research indicates that it exhibits a minimal inhibitory concentration (MIC) of 250 μg/ml against this pathogen, suggesting potential for use in aquaculture to control bacterial infections.
Mode of Action
The compound disrupts essential metabolic pathways within A. sobria, leading to bacterial death or growth inhibition. Its action may involve interference with cellular processes critical for the bacterium's survival.
Pharmacokinetics
Incorporation of deuterium into drug molecules like this compound can alter pharmacokinetic profiles, affecting absorption, distribution, metabolism, and excretion (ADME) characteristics. This property is particularly useful in tracing metabolic pathways in biological systems .
Interaction with Enzymes
This compound interacts significantly with various enzymes, notably phenylalanine ammonia-lyase (PAL), which catalyzes the conversion of phenylalanine to trans-cinnamic acid. This interaction is crucial for the biosynthesis of phenolic compounds essential for plant defense mechanisms and growth regulation.
Cellular Effects
In plant cells, this compound modulates cell signaling pathways and gene expression related to stress responses. This modulation enhances resistance to environmental stressors, indicating its role in plant metabolism and adaptation.
Antimicrobial Activity
Numerous studies have demonstrated the antimicrobial efficacy of this compound. For instance:
- Inhibition Studies : A study showed that varying concentrations of this compound significantly reduced bacterial viability, with a notable decrease observed at concentrations above 200 μg/ml .
- Comparative Efficacy : Compared to other cinnamic acid derivatives, this compound exhibited comparable or superior antimicrobial activity against various strains including Staphylococcus aureus and Escherichia coli with MIC values ranging from 12.5 to 50 μg/ml .
Pharmacological Studies
Research has also explored the pharmacological applications of this compound:
- Metabolic Tracer : Its stable isotope labeling makes it an excellent tracer in metabolic studies, allowing researchers to track its fate in biological systems .
- Cancer Research : Preliminary findings suggest potential anticancer properties, with some derivatives showing significant cytotoxicity against cancer cell lines such as MCF-7 (breast cancer) and HeLa (cervical cancer) at low concentrations .
Industrial Applications
In industrial settings, this compound is utilized in the synthesis of high-value chemicals and pharmaceuticals due to its unique properties. Its role as a precursor in various chemical processes underscores its economic significance .
Study on Antimicrobial Efficacy
A case study conducted by Chen et al. evaluated the antimicrobial effects of this compound against Mycobacterium tuberculosis. The study found that at concentrations ranging from 50 to 200 μg/ml, the compound exhibited substantial bactericidal activity, highlighting its potential as a natural antimicrobial agent .
Application in Plant Biology
Another study focused on the impact of this compound on plant stress responses. Results indicated enhanced resistance to drought conditions in treated plants compared to controls, suggesting applications in agricultural biotechnology for improving crop resilience.
Q & A
Basic Research Questions
Q. What are the critical physicochemical properties of trans-Cinnamic-d7 acid that influence experimental design?
- Answer: Key properties include its molecular formula (C₉HD₇O₂), molecular weight (155.20 g/mol), density (0.952 g/mL at 25°C), and melting point (132–135°C) . Stability under inert gas and avoidance of moisture/strong oxidizers are crucial for storage . Researchers should prioritize verifying these parameters using authoritative databases (e.g., NIST Chemistry WebBook) to ensure reproducibility .
Q. How is this compound synthesized, and what isotopic purity considerations apply?
- Answer: Synthesis typically involves deuterium exchange or incorporation during precursor reactions. For example, phenyl-d5 or deuterated acrylate intermediates may be used . Isotopic purity (e.g., 98 atom% D in phenyl-d5 derivatives) must be validated via mass spectrometry or NMR, with attention to avoiding proton contamination during handling .
Q. What analytical techniques are standard for characterizing this compound?
- Answer: Nuclear Magnetic Resonance (NMR) is critical for confirming deuterium placement and isotopic integrity. High-resolution mass spectrometry (HRMS) and Fourier-transform infrared spectroscopy (FTIR) validate molecular structure and functional groups. Cross-referencing with crystallographic data (e.g., SHELX-refined structures) ensures accuracy .
Advanced Research Questions
Q. How can this compound be optimized as a deuterated tracer in metabolic flux studies?
- Answer: Design experiments to track deuterium retention in target pathways. Use liquid chromatography-tandem mass spectrometry (LC-MS/MS) for high-sensitivity detection. Control for metabolic dilution effects by calibrating tracer concentrations against cell viability assays. Ensure compatibility with isotopically labeled internal standards .
Q. What methodologies address discrepancies in reported physicochemical data for this compound?
- Answer: Resolve conflicts by cross-referencing primary sources (e.g., NIST, SciFinder) and replicating measurements under standardized conditions . For contested properties like vapor pressure or solubility, employ controlled experiments (e.g., thermogravimetric analysis) and publish validation protocols to enhance dataset reliability .
Q. What strategies mitigate challenges in isotopic purity analysis during long-term stability studies?
- Answer: Implement accelerated stability testing under varied temperatures and humidity levels. Use quantitative NMR (qNMR) with deuterated solvents to monitor deuterium loss over time. Pair with kinetic isotope effect (KIE) studies to predict degradation pathways .
Q. How can computational tools enhance the application of this compound in structural biology?
- Answer: Integrate density functional theory (DFT) calculations to model deuterium’s impact on molecular interactions. Combine with X-ray crystallography (using SHELXL for refinement) to resolve deuterium positioning in protein-ligand complexes .
Q. Methodological Guidance
- Data Validation: Prioritize databases like NIST and SciFinder for physicochemical data, and use CTD for toxicogenomic insights .
- Experimental Reproducibility: Document isotopic batch variability and storage conditions explicitly in methods sections .
- Conflict Resolution: Publish negative results or validation studies to address literature gaps, adhering to citation standards in .
Properties
IUPAC Name |
(E)-2,3-dideuterio-3-(2,3,4,5,6-pentadeuteriophenyl)prop-2-enoic acid | |
---|---|---|
Source | PubChem | |
URL | https://pubchem.ncbi.nlm.nih.gov | |
Description | Data deposited in or computed by PubChem | |
InChI |
InChI=1S/C9H8O2/c10-9(11)7-6-8-4-2-1-3-5-8/h1-7H,(H,10,11)/b7-6+/i1D,2D,3D,4D,5D,6D,7D | |
Source | PubChem | |
URL | https://pubchem.ncbi.nlm.nih.gov | |
Description | Data deposited in or computed by PubChem | |
InChI Key |
WBYWAXJHAXSJNI-UJMUNGNDSA-N | |
Source | PubChem | |
URL | https://pubchem.ncbi.nlm.nih.gov | |
Description | Data deposited in or computed by PubChem | |
Canonical SMILES |
C1=CC=C(C=C1)C=CC(=O)O | |
Source | PubChem | |
URL | https://pubchem.ncbi.nlm.nih.gov | |
Description | Data deposited in or computed by PubChem | |
Isomeric SMILES |
[2H]C1=C(C(=C(C(=C1[2H])[2H])/C(=C(\[2H])/C(=O)O)/[2H])[2H])[2H] | |
Source | PubChem | |
URL | https://pubchem.ncbi.nlm.nih.gov | |
Description | Data deposited in or computed by PubChem | |
Molecular Formula |
C9H8O2 | |
Source | PubChem | |
URL | https://pubchem.ncbi.nlm.nih.gov | |
Description | Data deposited in or computed by PubChem | |
DSSTOX Substance ID |
DTXSID50584103 | |
Record name | (2E)-3-(~2~H_5_)Phenyl(~2~H_2_)prop-2-enoic acid | |
Source | EPA DSSTox | |
URL | https://comptox.epa.gov/dashboard/DTXSID50584103 | |
Description | DSSTox provides a high quality public chemistry resource for supporting improved predictive toxicology. | |
Molecular Weight |
155.20 g/mol | |
Source | PubChem | |
URL | https://pubchem.ncbi.nlm.nih.gov | |
Description | Data deposited in or computed by PubChem | |
CAS No. |
308796-47-6 | |
Record name | (2E)-3-(~2~H_5_)Phenyl(~2~H_2_)prop-2-enoic acid | |
Source | EPA DSSTox | |
URL | https://comptox.epa.gov/dashboard/DTXSID50584103 | |
Description | DSSTox provides a high quality public chemistry resource for supporting improved predictive toxicology. | |
Record name | trans-Cinnamic-d7 acid | |
Source | European Chemicals Agency (ECHA) | |
URL | https://echa.europa.eu/information-on-chemicals | |
Description | The European Chemicals Agency (ECHA) is an agency of the European Union which is the driving force among regulatory authorities in implementing the EU's groundbreaking chemicals legislation for the benefit of human health and the environment as well as for innovation and competitiveness. | |
Explanation | Use of the information, documents and data from the ECHA website is subject to the terms and conditions of this Legal Notice, and subject to other binding limitations provided for under applicable law, the information, documents and data made available on the ECHA website may be reproduced, distributed and/or used, totally or in part, for non-commercial purposes provided that ECHA is acknowledged as the source: "Source: European Chemicals Agency, http://echa.europa.eu/". Such acknowledgement must be included in each copy of the material. ECHA permits and encourages organisations and individuals to create links to the ECHA website under the following cumulative conditions: Links can only be made to webpages that provide a link to the Legal Notice page. | |
Synthesis routes and methods I
Procedure details
Synthesis routes and methods II
Procedure details
Synthesis routes and methods III
Procedure details
Synthesis routes and methods IV
Procedure details
Synthesis routes and methods V
Procedure details
Retrosynthesis Analysis
AI-Powered Synthesis Planning: Our tool employs the Template_relevance Pistachio, Template_relevance Bkms_metabolic, Template_relevance Pistachio_ringbreaker, Template_relevance Reaxys, Template_relevance Reaxys_biocatalysis model, leveraging a vast database of chemical reactions to predict feasible synthetic routes.
One-Step Synthesis Focus: Specifically designed for one-step synthesis, it provides concise and direct routes for your target compounds, streamlining the synthesis process.
Accurate Predictions: Utilizing the extensive PISTACHIO, BKMS_METABOLIC, PISTACHIO_RINGBREAKER, REAXYS, REAXYS_BIOCATALYSIS database, our tool offers high-accuracy predictions, reflecting the latest in chemical research and data.
Strategy Settings
Precursor scoring | Relevance Heuristic |
---|---|
Min. plausibility | 0.01 |
Model | Template_relevance |
Template Set | Pistachio/Bkms_metabolic/Pistachio_ringbreaker/Reaxys/Reaxys_biocatalysis |
Top-N result to add to graph | 6 |
Feasible Synthetic Routes
Disclaimer and Information on In-Vitro Research Products
Please be aware that all articles and product information presented on BenchChem are intended solely for informational purposes. The products available for purchase on BenchChem are specifically designed for in-vitro studies, which are conducted outside of living organisms. In-vitro studies, derived from the Latin term "in glass," involve experiments performed in controlled laboratory settings using cells or tissues. It is important to note that these products are not categorized as medicines or drugs, and they have not received approval from the FDA for the prevention, treatment, or cure of any medical condition, ailment, or disease. We must emphasize that any form of bodily introduction of these products into humans or animals is strictly prohibited by law. It is essential to adhere to these guidelines to ensure compliance with legal and ethical standards in research and experimentation.