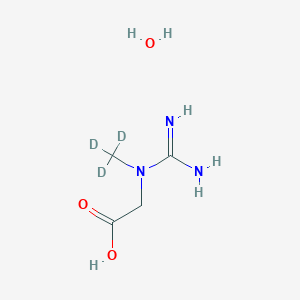
Creatine-(methyl-d3) monohydrate
Overview
Description
Creatine-(methyl-d3) monohydrate is a deuterium-labeled form of creatine monohydrate. It is a naturally occurring compound found in muscle tissue and is commonly used as a dietary supplement for athletes and bodybuilders. The deuterium labeling involves replacing the hydrogen atoms in the methyl group with deuterium, which is a stable isotope of hydrogen. This modification is often used in scientific research to trace metabolic pathways and study the pharmacokinetics of creatine.
Mechanism of Action
Target of Action
Creatine-(methyl-d3) monohydrate primarily targets Creatine Kinase enzymes, which include the M-type, U-type (mitochondrial), and B-type . These enzymes play a crucial role in energy metabolism in tissues with high and fluctuating energy demands, such as skeletal muscle and the brain .
Mode of Action
This compound interacts with its targets by binding to phosphate, forming phosphocreatine (PCr) . This reaction is catalyzed by Creatine Kinase . Phosphocreatine then binds with adenosine diphosphate (ADP) to convert it back to ATP (adenosine triphosphate), an important cellular energy source for short-term ATP needs prior to oxidative phosphorylation .
Biochemical Pathways
The primary biochemical pathway affected by this compound is the creatine-phosphocreatine pathway . This pathway plays a vital role in energy metabolism, particularly in tissues with high energy demands. The conversion of creatine to phosphocreatine and the subsequent regeneration of ATP from ADP are key steps in this pathway .
Pharmacokinetics
It is also known that insulin and insulin-stimulating foods can enhance muscle uptake of creatine .
Result of Action
The action of this compound results in the generation of ATP, providing energy for various cellular processes . This can enhance muscle function and exercise performance. Additionally, creatine may have neuroprotective and cardioprotective actions .
Action Environment
The action of this compound can be influenced by various environmental factors. For instance, high carbohydrate meals may slow the absorption of creatine from the intestine
Biochemical Analysis
Biochemical Properties
Creatine-(methyl-d3) monohydrate plays a crucial role in biochemical reactions involving energy transfer. It interacts with several enzymes, proteins, and other biomolecules. One of the primary interactions is with creatine kinase, an enzyme that catalyzes the conversion of creatine to phosphocreatine, a high-energy storage molecule. This reaction is essential for maintaining ATP levels in cells during periods of high energy demand . Additionally, this compound interacts with transport proteins such as the creatine transporter (CRT), which facilitates its uptake into cells .
Cellular Effects
This compound influences various cellular processes, including cell signaling pathways, gene expression, and cellular metabolism. In muscle cells, it enhances energy availability by increasing phosphocreatine levels, which in turn supports ATP regeneration during intense physical activity . In neuronal cells, this compound has been shown to improve cognitive function by supporting brain bioenergetics and reducing oxidative stress . These effects are mediated through its role in maintaining cellular energy homeostasis and modulating signaling pathways involved in cell survival and function .
Molecular Mechanism
At the molecular level, this compound exerts its effects through several mechanisms. It binds to creatine kinase, facilitating the formation of phosphocreatine from ATP and creatine . This reaction is critical for buffering cellular ATP levels, especially in tissues with high energy demands such as muscles and the brain. Additionally, this compound influences gene expression by modulating the activity of transcription factors and signaling molecules involved in energy metabolism and cellular stress responses .
Temporal Effects in Laboratory Settings
In laboratory settings, the effects of this compound can vary over time. Studies have shown that its stability and degradation are influenced by factors such as temperature and pH . Long-term exposure to this compound has been associated with sustained improvements in muscle mass and cognitive function, particularly in aging populations . These effects are likely due to the compound’s ability to maintain cellular energy homeostasis and reduce oxidative damage over extended periods .
Dosage Effects in Animal Models
The effects of this compound in animal models are dose-dependent. At lower doses, it has been shown to enhance muscle strength and endurance without adverse effects . At higher doses, there may be potential toxic effects, including renal stress and gastrointestinal disturbances . These findings highlight the importance of optimizing dosage to achieve the desired therapeutic effects while minimizing potential risks .
Metabolic Pathways
This compound is involved in several metabolic pathways, primarily those related to energy metabolism. It is synthesized endogenously through a two-step process involving the enzymes glycine amidinotransferase (GATM) and guanidinoacetate N-methyltransferase (GAMT) . Once synthesized, this compound is transported into cells via the creatine transporter (CRT) and converted to phosphocreatine by creatine kinase . This phosphocreatine serves as a readily available energy reservoir that can be rapidly mobilized to regenerate ATP during periods of high energy demand .
Transport and Distribution
Within cells, this compound is transported and distributed by the creatine transporter (CRT), which facilitates its uptake into tissues such as muscle and brain . Once inside the cells, it is distributed to various subcellular compartments, including the cytosol and mitochondria, where it participates in energy metabolism . The distribution of this compound is influenced by factors such as tissue type, metabolic activity, and the presence of specific transporters and binding proteins .
Subcellular Localization
This compound is localized in several subcellular compartments, including the cytosol, mitochondria, and sarcoplasmic reticulum . Its activity and function are influenced by its localization, with mitochondrial creatine playing a critical role in maintaining ATP levels during oxidative phosphorylation . Additionally, this compound may undergo post-translational modifications that affect its targeting to specific cellular compartments and its interactions with other biomolecules .
Preparation Methods
Synthetic Routes and Reaction Conditions
The synthesis of creatine-(methyl-d3) monohydrate typically involves the following steps:
Starting Materials: The synthesis begins with sarcosine and cyanamide.
Reaction Conditions: The reaction is carried out in an aqueous medium under controlled temperature and pH conditions.
Deuterium Labeling: Deuterium oxide (D2O) is used to replace the hydrogen atoms in the methyl group with deuterium.
Crystallization: The product is then crystallized to obtain this compound in high purity.
Industrial Production Methods
Industrial production of this compound follows similar steps but on a larger scale. The process involves:
Bulk Synthesis: Large quantities of sarcosine and cyanamide are reacted in industrial reactors.
Deuterium Exchange: Deuterium oxide is used in large volumes to ensure complete deuterium labeling.
Purification: The product is purified using crystallization and filtration techniques.
Quality Control: The final product undergoes rigorous quality control to ensure isotopic purity and chemical purity.
Chemical Reactions Analysis
Types of Reactions
Creatine-(methyl-d3) monohydrate undergoes several types of chemical reactions, including:
Oxidation: It can be oxidized to form creatinine-(methyl-d3).
Reduction: Under certain conditions, it can be reduced back to its precursor compounds.
Substitution: The deuterium atoms can be replaced with hydrogen under specific conditions.
Common Reagents and Conditions
Oxidation: Common oxidizing agents include potassium permanganate and hydrogen peroxide.
Reduction: Reducing agents such as sodium borohydride can be used.
Substitution: Acidic or basic conditions can facilitate the exchange of deuterium with hydrogen.
Major Products
Creatinine-(methyl-d3): Formed through oxidation.
Sarcosine and Cyanamide: Formed through reduction.
Creatine Monohydrate: Formed through deuterium-hydrogen exchange.
Scientific Research Applications
Creatine-(methyl-d3) monohydrate has a wide range of applications in scientific research:
Chemistry: Used as a tracer in metabolic studies to understand the pathways of creatine metabolism.
Biology: Helps in studying muscle physiology and energy metabolism.
Medicine: Used in pharmacokinetic studies to understand the absorption, distribution, metabolism, and excretion of creatine.
Industry: Employed in the development of dietary supplements and performance-enhancing products.
Comparison with Similar Compounds
Similar Compounds
Creatine Monohydrate: The non-labeled form of creatine.
Creatinine-(methyl-d3): The oxidized form of creatine-(methyl-d3).
Creatine Ethyl Ester: A derivative of creatine with an ethyl ester group.
Uniqueness
Creatine-(methyl-d3) monohydrate is unique due to its deuterium labeling, which makes it an invaluable tool for tracing metabolic pathways and studying the pharmacokinetics of creatine. This isotopic labeling provides insights that are not possible with non-labeled compounds, making it a critical compound in research settings.
Properties
IUPAC Name |
2-[carbamimidoyl(trideuteriomethyl)amino]acetic acid;hydrate | |
---|---|---|
Source | PubChem | |
URL | https://pubchem.ncbi.nlm.nih.gov | |
Description | Data deposited in or computed by PubChem | |
InChI |
InChI=1S/C4H9N3O2.H2O/c1-7(4(5)6)2-3(8)9;/h2H2,1H3,(H3,5,6)(H,8,9);1H2/i1D3; | |
Source | PubChem | |
URL | https://pubchem.ncbi.nlm.nih.gov | |
Description | Data deposited in or computed by PubChem | |
InChI Key |
MEJYXFHCRXAUIL-NIIDSAIPSA-N | |
Source | PubChem | |
URL | https://pubchem.ncbi.nlm.nih.gov | |
Description | Data deposited in or computed by PubChem | |
Canonical SMILES |
CN(CC(=O)O)C(=N)N.O | |
Source | PubChem | |
URL | https://pubchem.ncbi.nlm.nih.gov | |
Description | Data deposited in or computed by PubChem | |
Isomeric SMILES |
[2H]C([2H])([2H])N(CC(=O)O)C(=N)N.O | |
Source | PubChem | |
URL | https://pubchem.ncbi.nlm.nih.gov | |
Description | Data deposited in or computed by PubChem | |
Molecular Formula |
C4H11N3O3 | |
Source | PubChem | |
URL | https://pubchem.ncbi.nlm.nih.gov | |
Description | Data deposited in or computed by PubChem | |
DSSTOX Substance ID |
DTXSID20584150 | |
Record name | N-Carbamimidoyl-N-(~2~H_3_)methylglycine--water (1/1) | |
Source | EPA DSSTox | |
URL | https://comptox.epa.gov/dashboard/DTXSID20584150 | |
Description | DSSTox provides a high quality public chemistry resource for supporting improved predictive toxicology. | |
Molecular Weight |
152.17 g/mol | |
Source | PubChem | |
URL | https://pubchem.ncbi.nlm.nih.gov | |
Description | Data deposited in or computed by PubChem | |
CAS No. |
284664-86-4 | |
Record name | Glycine, N-(aminoiminomethyl)-N-(methyl-d3)-, hydrate (1:1) | |
Source | CAS Common Chemistry | |
URL | https://commonchemistry.cas.org/detail?cas_rn=284664-86-4 | |
Description | CAS Common Chemistry is an open community resource for accessing chemical information. Nearly 500,000 chemical substances from CAS REGISTRY cover areas of community interest, including common and frequently regulated chemicals, and those relevant to high school and undergraduate chemistry classes. This chemical information, curated by our expert scientists, is provided in alignment with our mission as a division of the American Chemical Society. | |
Explanation | The data from CAS Common Chemistry is provided under a CC-BY-NC 4.0 license, unless otherwise stated. | |
Record name | N-Carbamimidoyl-N-(~2~H_3_)methylglycine--water (1/1) | |
Source | EPA DSSTox | |
URL | https://comptox.epa.gov/dashboard/DTXSID20584150 | |
Description | DSSTox provides a high quality public chemistry resource for supporting improved predictive toxicology. | |
Record name | 284664-86-4 | |
Source | European Chemicals Agency (ECHA) | |
URL | https://echa.europa.eu/information-on-chemicals | |
Description | The European Chemicals Agency (ECHA) is an agency of the European Union which is the driving force among regulatory authorities in implementing the EU's groundbreaking chemicals legislation for the benefit of human health and the environment as well as for innovation and competitiveness. | |
Explanation | Use of the information, documents and data from the ECHA website is subject to the terms and conditions of this Legal Notice, and subject to other binding limitations provided for under applicable law, the information, documents and data made available on the ECHA website may be reproduced, distributed and/or used, totally or in part, for non-commercial purposes provided that ECHA is acknowledged as the source: "Source: European Chemicals Agency, http://echa.europa.eu/". Such acknowledgement must be included in each copy of the material. ECHA permits and encourages organisations and individuals to create links to the ECHA website under the following cumulative conditions: Links can only be made to webpages that provide a link to the Legal Notice page. | |
Synthesis routes and methods I
Procedure details
Synthesis routes and methods II
Procedure details
Synthesis routes and methods III
Procedure details
Synthesis routes and methods IV
Procedure details
Retrosynthesis Analysis
AI-Powered Synthesis Planning: Our tool employs the Template_relevance Pistachio, Template_relevance Bkms_metabolic, Template_relevance Pistachio_ringbreaker, Template_relevance Reaxys, Template_relevance Reaxys_biocatalysis model, leveraging a vast database of chemical reactions to predict feasible synthetic routes.
One-Step Synthesis Focus: Specifically designed for one-step synthesis, it provides concise and direct routes for your target compounds, streamlining the synthesis process.
Accurate Predictions: Utilizing the extensive PISTACHIO, BKMS_METABOLIC, PISTACHIO_RINGBREAKER, REAXYS, REAXYS_BIOCATALYSIS database, our tool offers high-accuracy predictions, reflecting the latest in chemical research and data.
Strategy Settings
Precursor scoring | Relevance Heuristic |
---|---|
Min. plausibility | 0.01 |
Model | Template_relevance |
Template Set | Pistachio/Bkms_metabolic/Pistachio_ringbreaker/Reaxys/Reaxys_biocatalysis |
Top-N result to add to graph | 6 |
Feasible Synthetic Routes
Disclaimer and Information on In-Vitro Research Products
Please be aware that all articles and product information presented on BenchChem are intended solely for informational purposes. The products available for purchase on BenchChem are specifically designed for in-vitro studies, which are conducted outside of living organisms. In-vitro studies, derived from the Latin term "in glass," involve experiments performed in controlled laboratory settings using cells or tissues. It is important to note that these products are not categorized as medicines or drugs, and they have not received approval from the FDA for the prevention, treatment, or cure of any medical condition, ailment, or disease. We must emphasize that any form of bodily introduction of these products into humans or animals is strictly prohibited by law. It is essential to adhere to these guidelines to ensure compliance with legal and ethical standards in research and experimentation.