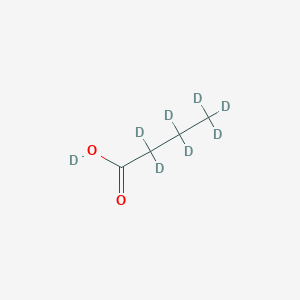
Butyric acid-d8
Overview
Description
Butyric acid-d8, also known as butanoic acid-d8, is a deuterated form of butyric acid. It is a short-chain fatty acid with the chemical formula CD3(CD2)2CO2D. The compound is characterized by the replacement of hydrogen atoms with deuterium, a stable isotope of hydrogen. This modification makes this compound particularly useful in various scientific research applications, especially in studies involving nuclear magnetic resonance (NMR) spectroscopy and mass spectrometry.
Mechanism of Action
Target of Action
Butyric acid-d8, a chemically stable isotope-labeled form of butyric acid, primarily targets the G protein-coupled receptors (GPCRs) and histone deacetylases . It is produced in the human body by the gut microbiota during the fermentation of dietary fibers . The compound’s primary targets play a crucial role in regulating host processes .
Mode of Action
This compound interacts with its targets, leading to significant changes in cellular processes. It inhibits the deacetylation and dephosphorylation of histones, making the DNA more accessible and ultimately improving transcription rates and cell-specific productivities . It also activates GPCRs, leading to the activation of downstream signaling pathways .
Biochemical Pathways
The main metabolic pathway of this compound involves the transformation of crotonyl CoA into butyryl CoA, which is then reduced to butyric acid . This process is catalyzed by various enzymes, including Beta-ketoacyl reductase . The production of butyric acid sets the stage for the subsequent generation of other fatty acids .
Pharmacokinetics
It is known that the compound can readily permeate the cytoplasm .
Result of Action
The action of this compound leads to molecular and cellular effects such as inhibition of cell proliferation and impact on histone modification . These translate into subsequently enhanced mechanisms of protein biosynthesis, regulation of transcription, messenger RNA processing and transport, ribosomal translation, and cellular trafficking of IgG intermediates .
Action Environment
The action, efficacy, and stability of this compound can be influenced by various environmental factors. For instance, the presence of dietary fibers in the gut environment can enhance the production of this compound by the gut microbiota
Biochemical Analysis
Biochemical Properties
Butyric acid-d8 shares similar biochemical properties with its unlabeled counterpart, butyric acid. It is a carboxylic acid with the chemical formula CD3(CD2)2CO2D . It readily donates a hydrogen ion, making it a weak acid . Butyric acid is produced naturally in various organisms, including bacteria, and can also be synthesized . It interacts with various enzymes, proteins, and other biomolecules, contributing to a range of biochemical reactions .
Cellular Effects
This compound, like butyric acid, plays a crucial role in cellular processes. It serves as an energy source for certain cells lining the gastrointestinal tract and has been linked to various health benefits . It influences cell function, including impact on cell signaling pathways, gene expression, and cellular metabolism . For instance, butyric acid has anti-inflammatory properties, which can help reduce inflammation in the gut and promote overall gut health .
Molecular Mechanism
The molecular mechanism of this compound involves its interactions with biomolecules, enzyme inhibition or activation, and changes in gene expression . It exerts its effects at the molecular level, including any binding interactions with biomolecules . As a histone deacetylase inhibitor, butyrate can stop the growth and induce cell death (called apoptosis) in colorectal cancer cells .
Temporal Effects in Laboratory Settings
In laboratory settings, the effects of butyrate change over time. This includes information on the product’s stability, degradation, and any long-term effects on cellular function observed in in vitro or in vivo studies . Butyrate exhibits a wide variety of biological activities in different pathways including energy homeostasis, glucose and lipid metabolism, inflammation, oxidative stress, neural signaling, and epigenetic modulation .
Dosage Effects in Animal Models
The effects of this compound vary with different dosages in animal models . For instance, in a 2007 animal study, after five weeks of treatment with butyric acid, obese mice lost 10.2 percent of their original body weight, and body fat was reduced by 10 percent .
Metabolic Pathways
This compound is involved in several metabolic pathways. It interacts with enzymes or cofactors and can affect metabolic flux or metabolite levels . Butyrate synthesis could be driven by coupling with an anaerobic energy conversion system .
Transport and Distribution
This compound is transported and distributed within cells and tissues . It could enter the portal vein and interact with various organs . The transport of this compound involves specific efflux carriers .
Subcellular Localization
It is known that butyrate and its conjugates can be transported in plants
Preparation Methods
Synthetic Routes and Reaction Conditions
Butyric acid-d8 can be synthesized through several methods. One common approach involves the deuteration of butyric acid using deuterium gas (D2) in the presence of a catalyst. This process typically requires high temperatures and pressures to facilitate the exchange of hydrogen atoms with deuterium. Another method involves the use of deuterated reagents, such as deuterated water (D2O) or deuterated organic solvents, in the synthesis of butyric acid.
Industrial Production Methods
Industrial production of this compound often involves the fermentation of deuterated substrates by specific bacterial strains, such as Clostridium tyrobutyricum. These bacteria can metabolize deuterated glucose or other deuterated carbohydrates to produce this compound. The fermentation process is typically carried out in bioreactors under anaerobic conditions, with careful control of pH, temperature, and nutrient supply to optimize yield and productivity .
Chemical Reactions Analysis
Types of Reactions
Butyric acid-d8 undergoes various chemical reactions typical of carboxylic acids. These include:
Oxidation: this compound can be oxidized to produce carbon dioxide and water.
Reduction: It can be reduced to butanol-d8 using reducing agents such as lithium aluminum hydride (LiAlH4).
Substitution: The carboxyl group can be substituted with other functional groups to form esters, amides, and anhydrides.
Common Reagents and Conditions
Oxidation: Common oxidizing agents include potassium permanganate (KMnO4) and chromium trioxide (CrO3).
Reduction: Reducing agents like LiAlH4 or catalytic hydrogenation using hydrogen gas (H2) and a metal catalyst (e.g., palladium on carbon).
Substitution: Esterification reactions typically use alcohols in the presence of an acid catalyst, such as sulfuric acid (H2SO4).
Major Products
Oxidation: Carbon dioxide (CO2) and water (H2O).
Reduction: Butanol-d8.
Substitution: Butyrate esters, butyramides, and butyric anhydride
Scientific Research Applications
Butyric acid-d8 is widely used in scientific research due to its unique properties. Some of its applications include:
Chemistry: Used as a tracer in NMR spectroscopy and mass spectrometry to study metabolic pathways and reaction mechanisms.
Biology: Employed in studies of gut microbiota and their metabolic products, as butyric acid is a key short-chain fatty acid produced by gut bacteria.
Medicine: Investigated for its potential therapeutic effects, including anti-inflammatory and anti-cancer properties. It is also used in studies of metabolic disorders and gastrointestinal health.
Industry: Utilized in the production of deuterated compounds for pharmaceuticals and other specialized chemical products
Comparison with Similar Compounds
Butyric acid-d8 can be compared with other deuterated and non-deuterated short-chain fatty acids:
Butyric acid: The non-deuterated form, with similar chemical properties but different applications due to the absence of deuterium.
Butyric-d7 acid: Another deuterated form with seven deuterium atoms, used for similar research purposes.
Hexanoic-d11 acid: A longer-chain deuterated fatty acid with different metabolic and chemical properties.
Propionic acid-d6: A shorter-chain deuterated fatty acid with distinct applications in metabolic studies.
This compound stands out due to its specific isotopic labeling, making it particularly valuable in studies requiring precise tracking of metabolic processes and molecular interactions.
Properties
IUPAC Name |
deuterio 2,2,3,3,4,4,4-heptadeuteriobutanoate | |
---|---|---|
Source | PubChem | |
URL | https://pubchem.ncbi.nlm.nih.gov | |
Description | Data deposited in or computed by PubChem | |
InChI |
InChI=1S/C4H8O2/c1-2-3-4(5)6/h2-3H2,1H3,(H,5,6)/i1D3,2D2,3D2/hD | |
Source | PubChem | |
URL | https://pubchem.ncbi.nlm.nih.gov | |
Description | Data deposited in or computed by PubChem | |
InChI Key |
FERIUCNNQQJTOY-FNQDQTMCSA-N | |
Source | PubChem | |
URL | https://pubchem.ncbi.nlm.nih.gov | |
Description | Data deposited in or computed by PubChem | |
Canonical SMILES |
CCCC(=O)O | |
Source | PubChem | |
URL | https://pubchem.ncbi.nlm.nih.gov | |
Description | Data deposited in or computed by PubChem | |
Isomeric SMILES |
[2H]C([2H])([2H])C([2H])([2H])C([2H])([2H])C(=O)O[2H] | |
Source | PubChem | |
URL | https://pubchem.ncbi.nlm.nih.gov | |
Description | Data deposited in or computed by PubChem | |
Molecular Formula |
C4H8O2 | |
Source | PubChem | |
URL | https://pubchem.ncbi.nlm.nih.gov | |
Description | Data deposited in or computed by PubChem | |
DSSTOX Substance ID |
DTXSID90584364 | |
Record name | (~2~H_7_)Butan(~2~H)oic acid | |
Source | EPA DSSTox | |
URL | https://comptox.epa.gov/dashboard/DTXSID90584364 | |
Description | DSSTox provides a high quality public chemistry resource for supporting improved predictive toxicology. | |
Molecular Weight |
96.15 g/mol | |
Source | PubChem | |
URL | https://pubchem.ncbi.nlm.nih.gov | |
Description | Data deposited in or computed by PubChem | |
CAS No. |
202468-80-2 | |
Record name | (~2~H_7_)Butan(~2~H)oic acid | |
Source | EPA DSSTox | |
URL | https://comptox.epa.gov/dashboard/DTXSID90584364 | |
Description | DSSTox provides a high quality public chemistry resource for supporting improved predictive toxicology. | |
Record name | 202468-80-2 | |
Source | European Chemicals Agency (ECHA) | |
URL | https://echa.europa.eu/information-on-chemicals | |
Description | The European Chemicals Agency (ECHA) is an agency of the European Union which is the driving force among regulatory authorities in implementing the EU's groundbreaking chemicals legislation for the benefit of human health and the environment as well as for innovation and competitiveness. | |
Explanation | Use of the information, documents and data from the ECHA website is subject to the terms and conditions of this Legal Notice, and subject to other binding limitations provided for under applicable law, the information, documents and data made available on the ECHA website may be reproduced, distributed and/or used, totally or in part, for non-commercial purposes provided that ECHA is acknowledged as the source: "Source: European Chemicals Agency, http://echa.europa.eu/". Such acknowledgement must be included in each copy of the material. ECHA permits and encourages organisations and individuals to create links to the ECHA website under the following cumulative conditions: Links can only be made to webpages that provide a link to the Legal Notice page. | |
Synthesis routes and methods I
Procedure details
Synthesis routes and methods II
Procedure details
Synthesis routes and methods III
Procedure details
Synthesis routes and methods IV
Procedure details
Retrosynthesis Analysis
AI-Powered Synthesis Planning: Our tool employs the Template_relevance Pistachio, Template_relevance Bkms_metabolic, Template_relevance Pistachio_ringbreaker, Template_relevance Reaxys, Template_relevance Reaxys_biocatalysis model, leveraging a vast database of chemical reactions to predict feasible synthetic routes.
One-Step Synthesis Focus: Specifically designed for one-step synthesis, it provides concise and direct routes for your target compounds, streamlining the synthesis process.
Accurate Predictions: Utilizing the extensive PISTACHIO, BKMS_METABOLIC, PISTACHIO_RINGBREAKER, REAXYS, REAXYS_BIOCATALYSIS database, our tool offers high-accuracy predictions, reflecting the latest in chemical research and data.
Strategy Settings
Precursor scoring | Relevance Heuristic |
---|---|
Min. plausibility | 0.01 |
Model | Template_relevance |
Template Set | Pistachio/Bkms_metabolic/Pistachio_ringbreaker/Reaxys/Reaxys_biocatalysis |
Top-N result to add to graph | 6 |
Feasible Synthetic Routes
Disclaimer and Information on In-Vitro Research Products
Please be aware that all articles and product information presented on BenchChem are intended solely for informational purposes. The products available for purchase on BenchChem are specifically designed for in-vitro studies, which are conducted outside of living organisms. In-vitro studies, derived from the Latin term "in glass," involve experiments performed in controlled laboratory settings using cells or tissues. It is important to note that these products are not categorized as medicines or drugs, and they have not received approval from the FDA for the prevention, treatment, or cure of any medical condition, ailment, or disease. We must emphasize that any form of bodily introduction of these products into humans or animals is strictly prohibited by law. It is essential to adhere to these guidelines to ensure compliance with legal and ethical standards in research and experimentation.