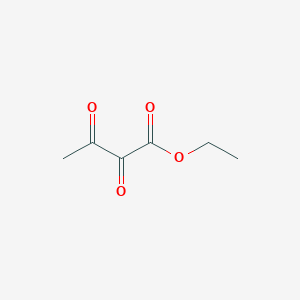
Ethyl 2,3-dioxobutanoate
Overview
Description
This compound serves as a versatile intermediate in organic synthesis, particularly in the preparation of heterocycles, pharmaceuticals, and agrochemicals. Its reactivity stems from the electron-withdrawing ketone groups, which enhance its participation in condensation, cyclization, and nucleophilic substitution reactions.
Preparation Methods
Synthetic Routes and Reaction Conditions: Ethyl 2,3-dioxobutanoate can be synthesized through several methods. One common approach involves the alkylation of enolate ions. The enolate ion, formed from the deprotonation of ethyl acetoacetate, reacts with an alkyl halide to form the desired product. The reaction typically occurs under basic conditions using sodium ethoxide in ethanol .
Industrial Production Methods: In industrial settings, the production of this compound often involves the esterification of butanedioic acid derivatives. The process includes the reaction of butanedioic acid with ethanol in the presence of a strong acid catalyst, such as sulfuric acid, to yield the ester product. The reaction is conducted under reflux conditions to ensure complete conversion.
Chemical Reactions Analysis
Types of Reactions: Ethyl 2,3-dioxobutanoate undergoes various chemical reactions, including:
Oxidation: The compound can be oxidized to form corresponding carboxylic acids.
Reduction: Reduction reactions can convert the oxo groups to hydroxyl groups, forming diols.
Substitution: Nucleophilic substitution reactions can occur at the carbonyl carbon, leading to the formation of substituted derivatives.
Common Reagents and Conditions:
Oxidation: Common oxidizing agents include potassium permanganate and chromium trioxide.
Reduction: Sodium borohydride and lithium aluminum hydride are frequently used reducing agents.
Substitution: Nucleophiles such as amines and alcohols can react with the carbonyl carbon under acidic or basic conditions.
Major Products:
Oxidation: Carboxylic acids.
Reduction: Diols.
Substitution: Substituted esters and amides.
Scientific Research Applications
Organic Synthesis
Ethyl 2,3-dioxobutanoate serves as a valuable building block in organic chemistry. Its structural features allow it to participate in a variety of reactions, leading to the synthesis of complex molecules.
1.1 Synthesis of Derivatives
- Hydrazone Derivatives : this compound can be reacted with aromatic amines to form hydrazone derivatives, which have shown potential in biological activity. For instance, the synthesis of ethyl 2-[(3-chlorophenyl)hydrazono]-3-oxobutanoate demonstrated notable cytotoxic properties against certain cancer cell lines .
- Asymmetric Reduction : Research has highlighted the asymmetric reduction of ethyl 2-methyl-3-oxobutanoate using Chlorella species, leading to the production of enantiomerically enriched alcohols. This process is significant for synthesizing chiral compounds used in pharmaceuticals .
Drug Delivery Systems
Recent studies have explored the incorporation of this compound into mesoporous materials for drug delivery applications.
2.1 Mesoporous Silica Nanoparticles
- Synthesis and Functionality : this compound has been utilized in the synthesis of functionalized mesoporous silica nanoparticles (MSNs). These nanoparticles can be modified to enhance drug loading capacity and release profiles. For example, a study demonstrated that MSNs coated with ethyl derivatives effectively encapsulated and released anticancer drugs in a controlled manner .
Material | Drug Loading (%) | Release Rate (%) | Application |
---|---|---|---|
Ethyl-coated MSNs | 75 | 60 | Anticancer drug delivery |
Non-coated MSNs | 50 | 30 | Standard drug delivery |
Environmental Remediation
This compound plays a role in environmental science, particularly in the removal of heavy metals from wastewater.
3.1 Adsorption Studies
- Heavy Metal Removal : The compound has been investigated as part of composite materials designed for heavy metal adsorption. Studies have shown that materials modified with ethyl derivatives exhibit enhanced adsorption capacities for metals such as cadmium (Cd²⁺) and lead (Pb²⁺). The optimization of these materials has been conducted using response surface methodology (RSM) .
Metal Ion | Adsorption Capacity (mg/g) | Material Used |
---|---|---|
Cd²⁺ | 150 | Ethyl-modified clay composites |
Pb²⁺ | 120 | Ethyl-functionalized silica |
Mechanism of Action
The mechanism of action of ethyl 2,3-dioxobutanoate involves its reactivity as an electrophile due to the presence of carbonyl groups. These groups can undergo nucleophilic attack, leading to the formation of various intermediates and products. The compound’s molecular targets include nucleophiles such as amines, alcohols, and thiols, which can react with the carbonyl carbon to form new bonds and structures.
Comparison with Similar Compounds
Structural and Functional Group Analysis
The table below compares Ethyl 2,3-dioxobutanoate with structurally related esters, emphasizing substituents, molecular weights, and functional groups:
*Hypothetical structure inferred from evidence on analogous compounds.
Key Observations :
- Substituent Effects: Halogenation (e.g., Cl in , Br in ) increases molecular weight and reactivity, favoring electrophilic substitutions. Aromatic moieties (e.g., methoxyphenyl in ) enable π-π interactions, critical in pharmaceutical applications.
Biological Activity
Ethyl 2,3-dioxobutanoate, a compound belonging to the class of β-keto esters, has garnered attention for its diverse biological activities. This article explores its pharmacological properties, including antimicrobial, anthelmintic, and cytotoxic effects, supported by data from various studies.
Chemical Structure and Properties
This compound is characterized by its β-dicarbonyl structure, which is essential for its biological activity. The compound can be synthesized through various methods, including the reaction of ethyl acetoacetate with appropriate reagents. Its structure facilitates interactions with biological targets, influencing its pharmacological effects.
Biological Activities
1. Antimicrobial Activity
Recent studies have demonstrated the antimicrobial potential of this compound derivatives. For instance, a study reported that certain derivatives exhibited significant antibacterial activity against multidrug-resistant strains of bacteria such as Escherichia coli and Staphylococcus aureus. The minimum inhibitory concentration (MIC) values for these compounds ranged from 0.073 to 0.125 mg/ml, indicating potent antibacterial properties .
Table 1: Antibacterial Activity of this compound Derivatives
Compound | MIC (mg/ml) against E. coli | MIC (mg/ml) against S. aureus |
---|---|---|
Compound 1 | 0.125 | 0.109 |
Compound 2 | 0.083 | 0.073 |
2. Anthelmintic Activity
This compound has also shown promising anthelmintic activity. Research indicated that certain derivatives could effectively paralyze and kill helminths such as Pheretima posthuma. The time to paralysis and death was significantly reduced in treated specimens compared to controls.
Table 2: Anthelmintic Activity of this compound Derivatives
Concentration (mg/ml) | Paralysis Time (min) | Death Time (min) |
---|---|---|
Compound A (5) | 10.50 ± 2.29 | 41.33 ± 1.52 |
Compound A (10) | 7.33 ± 2.56 | 20.66 ± 1.15 |
Albendazole (5) | 12.66 ± 1.52 | 49.67 ± 1.52 |
3. Cytotoxicity
The cytotoxic effects of this compound derivatives have been evaluated in various cancer cell lines. One study found that certain derivatives exhibited cytotoxicity comparable to established chemotherapeutics like etoposide, with lethal concentration (LC50) values ranging from 280 to 765 µg/ml .
The biological activity of this compound is attributed to its ability to interact with specific enzymes and receptors within microbial and mammalian cells:
- Antimicrobial Action : The β-dicarbonyl moiety is known to chelate metal ions essential for microbial growth.
- Anthelmintic Mechanism : These compounds may disrupt the neuromuscular function in helminths.
- Cytotoxic Effects : The compounds may induce apoptosis in cancer cells through the activation of intrinsic pathways.
Case Studies
Case Study: Antimicrobial Efficacy Against MDR Pathogens
A recent study investigated the efficacy of ethyl dioxobutanoate derivatives against multidrug-resistant pathogens in vitro. The findings revealed that these compounds not only inhibited bacterial growth but also showed synergistic effects when combined with conventional antibiotics.
Case Study: Anthelmintic Activity in Animal Models
Another study assessed the anthelmintic properties of ethyl dioxobutanoate derivatives in vivo using a rat model infected with helminths. Results indicated a significant reduction in parasite load and improved health outcomes for treated animals.
Q & A
Q. What are the established synthetic routes for Ethyl 2,3-dioxobutanoate, and what analytical techniques are recommended for its characterization?
Basic Research Question
this compound is typically synthesized via transesterification or condensation reactions. For example, analogous dioxobutanoate esters are prepared using reagents like ethyl chloro-oxoacetate, triethylamine, and DMAP in dichloromethane, followed by purification via column chromatography (SiO₂, pentane:EtOAc) . Characterization relies on spectral studies (¹H/¹³C NMR, IR) and elemental analysis to confirm structural integrity. Mass spectrometry (exact mass: 144.042 g/mol) and IUPAC nomenclature validation are critical for unambiguous identification .
Q. What spectroscopic markers (NMR, IR) are most diagnostic for confirming the structure of this compound?
Basic Research Question
Key spectroscopic features include:
- ¹H NMR : Signals for the ethyl ester group (δ ~1.3 ppm for CH₃, δ ~4.2 ppm for CH₂), coupled with keto-enol tautomerism peaks (δ ~3.5–5.5 ppm for α-protons to carbonyl groups) .
- IR : Strong absorption bands at ~1740 cm⁻¹ (ester C=O) and ~1700 cm⁻¹ (keto C=O) .
- MS : Molecular ion peak at m/z 144.042 (C₆H₈O₄) .
Q. What are the optimal storage conditions for this compound to maintain its stability in laboratory settings?
Basic Research Question
this compound should be stored at +4°C in airtight, light-resistant containers to prevent degradation via hydrolysis or photochemical reactions. Stability is enhanced by minimizing exposure to moisture and oxygen . Purity checks via HPLC or GC-MS are recommended before use in sensitive reactions .
Q. In what ways does this compound serve as a key intermediate in the synthesis of bioactive heterocyclic compounds?
Advanced Research Question
The compound’s α,β-diketo ester functionality enables its use in cyclocondensation reactions with hydrazines or hydroxylamine to form pyrazoles and isoxazoles, respectively. For example, condensation with phenyl hydrazine yields 5-(benzofuran-2-yl)-1-phenyl-1H-pyrazole-3-carboxylate derivatives, which exhibit antimicrobial activity . Fluorinated analogs (e.g., ethyl 4-(5-fluoropyridin-2-yl)-2,4-dioxobutanoate) are also synthesized for structure-activity relationship studies in drug discovery .
Q. How can researchers address discrepancies in reported reaction yields when using this compound as a precursor?
Advanced Research Question
Yield variations often arise from differences in reaction conditions (e.g., catalyst loading, solvent polarity, or temperature). Methodological approaches include:
- Statistical Analysis : Design of Experiments (DoE) to identify critical variables .
- Purity Control : Use of freshly distilled reagents and anhydrous solvents to minimize side reactions .
- Replication : Independent replication of reported protocols with detailed documentation of deviations .
Q. How does the electronic configuration of this compound influence its reactivity in nucleophilic addition reactions?
Advanced Research Question
The electron-withdrawing keto groups activate the α-carbon toward nucleophilic attack, facilitating reactions with amines or hydrazines. Computational studies (e.g., DFT) can model charge distribution and predict regioselectivity in cyclization reactions. Experimental validation via kinetic isotope effects or Hammett plots further elucidates mechanistic pathways .
Q. What strategies are recommended for resolving contradictions in spectral data interpretations of this compound derivatives?
Advanced Research Question
Contradictions often stem from tautomeric equilibria or solvent effects. Strategies include:
- Variable Temperature NMR : To observe tautomer interconversion .
- Cross-Validation : Comparing data from multiple techniques (e.g., X-ray crystallography for solid-state structure vs. solution NMR) .
- Collaborative Analysis : Peer review with computational chemists to align experimental and theoretical results .
Q. What role does this compound play in the development of fluorinated pharmaceutical intermediates?
Advanced Research Question
Fluorinated derivatives (e.g., ethyl 4-(5-fluoropyridin-2-yl)-2,4-dioxobutanoate) are synthesized via nucleophilic aromatic substitution or transition-metal-catalyzed coupling. These compounds serve as precursors to fluorinated pyrazoles, which are explored for enhanced metabolic stability and bioavailability in drug candidates .
Properties
IUPAC Name |
ethyl 2,3-dioxobutanoate | |
---|---|---|
Source | PubChem | |
URL | https://pubchem.ncbi.nlm.nih.gov | |
Description | Data deposited in or computed by PubChem | |
InChI |
InChI=1S/C6H8O4/c1-3-10-6(9)5(8)4(2)7/h3H2,1-2H3 | |
Source | PubChem | |
URL | https://pubchem.ncbi.nlm.nih.gov | |
Description | Data deposited in or computed by PubChem | |
InChI Key |
TYEPWEZRMUOMJT-UHFFFAOYSA-N | |
Source | PubChem | |
URL | https://pubchem.ncbi.nlm.nih.gov | |
Description | Data deposited in or computed by PubChem | |
Canonical SMILES |
CCOC(=O)C(=O)C(=O)C | |
Source | PubChem | |
URL | https://pubchem.ncbi.nlm.nih.gov | |
Description | Data deposited in or computed by PubChem | |
Molecular Formula |
C6H8O4 | |
Source | PubChem | |
URL | https://pubchem.ncbi.nlm.nih.gov | |
Description | Data deposited in or computed by PubChem | |
DSSTOX Substance ID |
DTXSID10482873 | |
Record name | ethyl 2,3-dioxobutanoate | |
Source | EPA DSSTox | |
URL | https://comptox.epa.gov/dashboard/DTXSID10482873 | |
Description | DSSTox provides a high quality public chemistry resource for supporting improved predictive toxicology. | |
Molecular Weight |
144.12 g/mol | |
Source | PubChem | |
URL | https://pubchem.ncbi.nlm.nih.gov | |
Description | Data deposited in or computed by PubChem | |
CAS No. |
1723-25-7 | |
Record name | ethyl 2,3-dioxobutanoate | |
Source | EPA DSSTox | |
URL | https://comptox.epa.gov/dashboard/DTXSID10482873 | |
Description | DSSTox provides a high quality public chemistry resource for supporting improved predictive toxicology. | |
Synthesis routes and methods
Procedure details
Retrosynthesis Analysis
AI-Powered Synthesis Planning: Our tool employs the Template_relevance Pistachio, Template_relevance Bkms_metabolic, Template_relevance Pistachio_ringbreaker, Template_relevance Reaxys, Template_relevance Reaxys_biocatalysis model, leveraging a vast database of chemical reactions to predict feasible synthetic routes.
One-Step Synthesis Focus: Specifically designed for one-step synthesis, it provides concise and direct routes for your target compounds, streamlining the synthesis process.
Accurate Predictions: Utilizing the extensive PISTACHIO, BKMS_METABOLIC, PISTACHIO_RINGBREAKER, REAXYS, REAXYS_BIOCATALYSIS database, our tool offers high-accuracy predictions, reflecting the latest in chemical research and data.
Strategy Settings
Precursor scoring | Relevance Heuristic |
---|---|
Min. plausibility | 0.01 |
Model | Template_relevance |
Template Set | Pistachio/Bkms_metabolic/Pistachio_ringbreaker/Reaxys/Reaxys_biocatalysis |
Top-N result to add to graph | 6 |
Feasible Synthetic Routes
Disclaimer and Information on In-Vitro Research Products
Please be aware that all articles and product information presented on BenchChem are intended solely for informational purposes. The products available for purchase on BenchChem are specifically designed for in-vitro studies, which are conducted outside of living organisms. In-vitro studies, derived from the Latin term "in glass," involve experiments performed in controlled laboratory settings using cells or tissues. It is important to note that these products are not categorized as medicines or drugs, and they have not received approval from the FDA for the prevention, treatment, or cure of any medical condition, ailment, or disease. We must emphasize that any form of bodily introduction of these products into humans or animals is strictly prohibited by law. It is essential to adhere to these guidelines to ensure compliance with legal and ethical standards in research and experimentation.