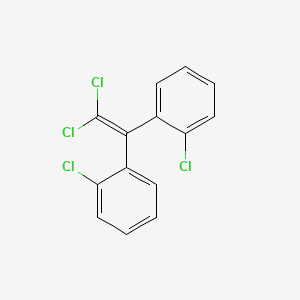
o,o'-DDE
Overview
Description
o,o’-Dichlorodiphenyldichloroethylene (o,o’-DDE) is a degradation product of dichlorodiphenyltrichloroethane (DDT), a well-known insecticide. o,o’-DDE is an organochlorine compound that is persistent in the environment and can accumulate in the tissues of living organisms. It is known for its stability and resistance to degradation, which contributes to its long-term environmental presence .
Preparation Methods
o,o’-DDE is primarily formed through the environmental degradation of DDT. The degradation process involves the removal of hydrogen chloride (HCl) from DDT, resulting in the formation of DDE. This process can occur under various environmental conditions, including exposure to sunlight and microbial activity .
Chemical Reactions Analysis
o,o’-DDE undergoes several types of chemical reactions, including:
Oxidation: o,o’-DDE can be oxidized to form other degradation products. Common oxidizing agents include potassium permanganate (KMnO4) and hydrogen peroxide (H2O2).
Reduction: Reduction of o,o’-DDE can lead to the formation of less chlorinated compounds. Reducing agents such as sodium borohydride (NaBH4) are often used in these reactions.
Substitution: o,o’-DDE can undergo substitution reactions where chlorine atoms are replaced by other functional groups.
Scientific Research Applications
o,o’-DDE has been extensively studied for its environmental impact and its role as a persistent organic pollutant. It is used in research to understand the long-term effects of organochlorine compounds on ecosystems and human health. Studies have shown that o,o’-DDE can accumulate in the food chain, leading to potential health risks for top predators, including humans .
In addition to environmental research, o,o’-DDE is also used in toxicological studies to assess its effects on various biological systems. It has been shown to have endocrine-disrupting properties, affecting hormone regulation in both wildlife and humans .
Mechanism of Action
o,o’-DDE exerts its effects primarily through its interaction with the endocrine system. It can mimic or interfere with the action of natural hormones, leading to disruptions in hormone signaling pathways. o,o’-DDE has been shown to bind to estrogen receptors, altering the expression of estrogen-responsive genes. This can result in reproductive and developmental abnormalities in exposed organisms .
Comparison with Similar Compounds
o,o’-DDE is similar to other degradation products of DDT, such as p,p’-DDE and p,p’-DDD. o,o’-DDE is unique in its specific chemical structure and its persistence in the environment. Compared to p,p’-DDE, o,o’-DDE is less commonly studied but still poses significant environmental and health risks .
Similar compounds include:
p,p’-DDE: Another degradation product of DDT, known for its persistence and bioaccumulation.
Conclusion
o,o’-DDE is a significant environmental pollutant with persistent and bioaccumulative properties. Its stability and resistance to degradation make it a compound of interest in environmental and toxicological research. Understanding its chemical behavior, environmental impact, and health effects is crucial for developing strategies to mitigate its presence in the environment.
Properties
IUPAC Name |
1-chloro-2-[2,2-dichloro-1-(2-chlorophenyl)ethenyl]benzene | |
---|---|---|
Source | PubChem | |
URL | https://pubchem.ncbi.nlm.nih.gov | |
Description | Data deposited in or computed by PubChem | |
InChI |
InChI=1S/C14H8Cl4/c15-11-7-3-1-5-9(11)13(14(17)18)10-6-2-4-8-12(10)16/h1-8H | |
Source | PubChem | |
URL | https://pubchem.ncbi.nlm.nih.gov | |
Description | Data deposited in or computed by PubChem | |
InChI Key |
KXIMKQKQRACGHT-UHFFFAOYSA-N | |
Source | PubChem | |
URL | https://pubchem.ncbi.nlm.nih.gov | |
Description | Data deposited in or computed by PubChem | |
Canonical SMILES |
C1=CC=C(C(=C1)C(=C(Cl)Cl)C2=CC=CC=C2Cl)Cl | |
Source | PubChem | |
URL | https://pubchem.ncbi.nlm.nih.gov | |
Description | Data deposited in or computed by PubChem | |
Molecular Formula |
C14H8Cl4 | |
Source | PubChem | |
URL | https://pubchem.ncbi.nlm.nih.gov | |
Description | Data deposited in or computed by PubChem | |
Molecular Weight |
318.0 g/mol | |
Source | PubChem | |
URL | https://pubchem.ncbi.nlm.nih.gov | |
Description | Data deposited in or computed by PubChem | |
CAS No. |
3328-98-1 | |
Record name | O,O'-Dde | |
Source | ChemIDplus | |
URL | https://pubchem.ncbi.nlm.nih.gov/substance/?source=chemidplus&sourceid=0003328981 | |
Description | ChemIDplus is a free, web search system that provides access to the structure and nomenclature authority files used for the identification of chemical substances cited in National Library of Medicine (NLM) databases, including the TOXNET system. | |
Record name | O,O'-DDE | |
Source | FDA Global Substance Registration System (GSRS) | |
URL | https://gsrs.ncats.nih.gov/ginas/app/beta/substances/151Q6M24OJ | |
Description | The FDA Global Substance Registration System (GSRS) enables the efficient and accurate exchange of information on what substances are in regulated products. Instead of relying on names, which vary across regulatory domains, countries, and regions, the GSRS knowledge base makes it possible for substances to be defined by standardized, scientific descriptions. | |
Explanation | Unless otherwise noted, the contents of the FDA website (www.fda.gov), both text and graphics, are not copyrighted. They are in the public domain and may be republished, reprinted and otherwise used freely by anyone without the need to obtain permission from FDA. Credit to the U.S. Food and Drug Administration as the source is appreciated but not required. | |
Synthesis routes and methods I
Procedure details
Synthesis routes and methods II
Procedure details
Synthesis routes and methods III
Procedure details
Synthesis routes and methods IV
Procedure details
Retrosynthesis Analysis
AI-Powered Synthesis Planning: Our tool employs the Template_relevance Pistachio, Template_relevance Bkms_metabolic, Template_relevance Pistachio_ringbreaker, Template_relevance Reaxys, Template_relevance Reaxys_biocatalysis model, leveraging a vast database of chemical reactions to predict feasible synthetic routes.
One-Step Synthesis Focus: Specifically designed for one-step synthesis, it provides concise and direct routes for your target compounds, streamlining the synthesis process.
Accurate Predictions: Utilizing the extensive PISTACHIO, BKMS_METABOLIC, PISTACHIO_RINGBREAKER, REAXYS, REAXYS_BIOCATALYSIS database, our tool offers high-accuracy predictions, reflecting the latest in chemical research and data.
Strategy Settings
Precursor scoring | Relevance Heuristic |
---|---|
Min. plausibility | 0.01 |
Model | Template_relevance |
Template Set | Pistachio/Bkms_metabolic/Pistachio_ringbreaker/Reaxys/Reaxys_biocatalysis |
Top-N result to add to graph | 6 |
Feasible Synthetic Routes
Disclaimer and Information on In-Vitro Research Products
Please be aware that all articles and product information presented on BenchChem are intended solely for informational purposes. The products available for purchase on BenchChem are specifically designed for in-vitro studies, which are conducted outside of living organisms. In-vitro studies, derived from the Latin term "in glass," involve experiments performed in controlled laboratory settings using cells or tissues. It is important to note that these products are not categorized as medicines or drugs, and they have not received approval from the FDA for the prevention, treatment, or cure of any medical condition, ailment, or disease. We must emphasize that any form of bodily introduction of these products into humans or animals is strictly prohibited by law. It is essential to adhere to these guidelines to ensure compliance with legal and ethical standards in research and experimentation.