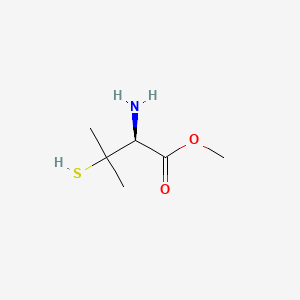
Penicillamine methyl ester
Overview
Description
Penicillamine methyl ester (Pme) is a derivative of penicillamine (β,β-dimethylcysteine), where the carboxylic acid group is replaced by a methyl ester. Pme acts as a tetradentate ligand, coordinating metal ions through its NH₂, SH, and C=O groups, forming stable complexes with lanthanides and transition metals . It is utilized in diverse applications, including metal chelation therapy, peroxynitrite scavenging, and as a precursor in organic synthesis .
Preparation Methods
Esterification of Penicillamine
The most common and direct method for preparing penicillamine methyl ester is the esterification of penicillamine with methanol in the presence of an acid catalyst.
- Starting Material: Penicillamine (either natural or synthetic, including dl-penicillamine)
- Reagents: Methanol (anhydrous), acid catalyst (commonly sulfuric acid or hydrogen chloride)
- Conditions: Reflux under anhydrous conditions for extended periods (typically 40–45 hours)
- Workup: Removal of water formed during the reaction, neutralization, extraction, and purification by recrystallization or distillation
$$
\text{Penicillamine} + \text{Methanol} \xrightarrow[\text{Reflux}]{\text{H}2\text{SO}4 \text{ or HCl}} \text{this compound} + \text{Water}
$$
This method is scalable for industrial production, where temperature, stirring, and solvent removal are carefully controlled to maximize yield and purity.
Preparation of this compound Hydrochloride Intermediate
A key intermediate in the synthesis is the this compound hydrochloride, which is crystalline and easier to handle.
- Dissolve penicillamine hydrochloride in absolute methanol saturated with anhydrous hydrogen chloride.
- Reflux the mixture for 40 hours.
- Concentrate the solution and induce crystallization by adding anhydrous ether.
- Collect and dry the crystalline ester hydrochloride.
- Dissolve the ester hydrochloride in water.
- Neutralize with sodium bicarbonate.
- Extract the free ester with chloroform.
- Dry over anhydrous sodium sulfate.
- Remove solvent under reduced pressure to obtain this compound.
This method yields crystalline methyl ester hydrochloride with melting points reported around 163–168 °C (with decomposition), indicating good purity.
Condensation with Oxazolone Derivatives
Another method involves condensation of this compound with 2-substituted oxazolone derivatives to form penicillenate esters, which are chemically related compounds.
- Equimolecular quantities of d-penicillamine methyl ester are condensed with 2-benzyl-4-methoxymethylene-5(4)-oxazolone.
- The reaction is carried out in organic solvents such as toluene or benzene.
- Water or alcohol formed during the reaction is continuously removed to drive the reaction forward.
- The product is recovered by standard isolation techniques.
This method allows the synthesis of various penicillenate esters by varying the oxazolone substituents and is useful for preparing intermediates for further chemical transformations.
Synthetic Routes from Penicillin Degradation or dl-Valine
Penicillamine itself can be prepared synthetically from penicillin degradation or from dl-valine via intermediates such as 2-methyl-4-isopropylidene-5(4)-oxazolone. These routes provide the penicillamine starting material for subsequent esterification.
- Penicillamine is obtained by hydrolyzing penicillin with hot dilute mineral acid.
- Alternatively, synthetic dl-penicillamine is prepared via acetylation, oxazolone formation, and hydrogen sulfide treatment.
- The penicillamine is then esterified as described above.
These methods ensure a steady supply of penicillamine for methyl ester preparation.
Data Table: Summary of Preparation Methods
Analytical and Purification Techniques
- Purification: Recrystallization from ethyl acetate or ether; distillation under reduced pressure.
- Characterization: Melting point determination, typically 163–168 °C for methyl ester hydrochloride.
- Extraction: Use of chloroform and drying agents like anhydrous sodium sulfate.
- Yield Optimization: Removal of water or alcohol formed during esterification or condensation reactions is critical to drive the reaction to completion.
Summary of Key Research Findings
- The esterification of penicillamine with methanol under acidic conditions is the most straightforward and industrially viable method.
- Formation of this compound hydrochloride as an isolable intermediate facilitates handling and purification.
- Condensation reactions with oxazolone derivatives expand the chemical utility of this compound for synthesizing related compounds.
- Synthetic routes to penicillamine from penicillin degradation or dl-valine ensure availability of the starting amino acid.
- Reaction parameters such as solvent choice (toluene, benzene), temperature, and removal of by-products significantly influence yield and purity.
- Crystalline ester hydrochlorides exhibit distinct melting points, serving as quality control markers.
This detailed analysis consolidates the preparation methods of this compound from multiple authoritative patent documents and chemical literature, providing a comprehensive guide for researchers and industrial chemists engaged in the synthesis of this important compound.
Chemical Reactions Analysis
Esterification and Thioesterification
S-Methylation
In metabolic pathways, the thiol group undergoes methylation:
textD-Penicillamine → S-Methyl-D-penicillamine Enzyme: Thiol methyltransferase (in vivo)[5]. Median urinary excretion (Parkinson’s patients): 177% higher than controls[5].
Hydrolysis Reactions
Acidic or basic conditions cleave the ester group, regenerating penicillamine:
textPenicillamine methyl ester + HCl → Penicillamine hydrochloride Conditions: Reflux with concentrated HCl (overnight)[2]. Yield: 79% (hydrochloride salt); 81% after recrystallization[2].
Chelation and Coordination Chemistry
This compound forms stable complexes with lanthanides (Ln³⁺) via NH₂, SH, and carbonyl groups .
Table 1: Formation Constants (log β) of Ln³⁺ Complexes
Lanthanide | log β (ML) | log β (ML₂) |
---|---|---|
La³⁺ | 4.50 | 8.84 |
Gd³⁺ | 4.75 | 9.21 |
Yb³⁺ | 4.62 | 8.99 |
Key observations :
-
Stability constants increase with lanthanide atomic number.
Metabolic Pathways
In humans, this compound undergoes:
-
S-Methylation : Major pathway mediated by thiol methyltransferase .
-
N-Acetylation and disulfide formation : Minor metabolic routes .
Table 2: Urinary Metabolite Levels (8-h collection)
Population | S-Methyl-D-penicillamine (µmol) |
---|---|
Healthy controls | 12.4 |
Parkinson’s | 34.3 |
Motor Neurone | 38.1 |
Stability and Degradation
Scientific Research Applications
Chemistry
Penicillamine methyl ester serves as a building block in organic synthesis. Its ability to act as a ligand in coordination chemistry facilitates the formation of metal complexes, which are essential in catalysis and material science.
Biology
Research indicates that this compound can modulate biological pathways due to its structural similarity to cysteine. Studies have shown that it can inhibit macrophage activity and reduce interleukin-1 (IL-1) levels, impacting immune responses and inflammation .
Medicine
The compound is primarily investigated for its therapeutic potential in treating conditions such as:
- Wilson's Disease : this compound binds copper ions, promoting their excretion through urine, thereby reducing copper toxicity in affected individuals.
- Cystinuria : Recent studies suggest that related compounds like L-cystine dimethyl ester (CDME), which share structural similarities with this compound, effectively inhibit cystine stone formation in animal models .
Case Study 1: Wilson's Disease Treatment
A clinical study demonstrated that patients treated with penicillamine experienced significant reductions in serum copper levels and improved liver function tests. The compound's efficacy was attributed to its chelating properties, facilitating copper elimination from the body.
Case Study 2: Cystinuria-Induced Urolithiasis
In a knockout mouse model of cystinuria, treatment with CDME showed a marked decrease in stone size compared to controls. This suggests that compounds related to this compound may offer new avenues for managing cystine stone formation without disrupting cystine metabolism .
Industrial Applications
In the pharmaceutical industry, this compound is utilized as an intermediate in synthesizing various drugs. Its role as a chelating agent extends to applications in detoxifying heavy metals from biological systems, making it valuable for developing treatments for heavy metal poisoning.
Mechanism of Action
Penicillamine methyl ester exerts its effects primarily through its thiol group, which can chelate metal ions and participate in redox reactions. The molecular targets include:
Metal Ions: Chelates copper, lead, and mercury, facilitating their excretion from the body.
Enzymes: Inhibits enzymes that require metal cofactors by binding to the metal ions.
Proteins: Interacts with cysteine residues in proteins, affecting their structure and function.
Comparison with Similar Compounds
Chemical and Coordination Properties
Table 1: Key Chemical Properties
Compound | Functional Groups | Metal Coordination Sites | Lipophilicity |
---|---|---|---|
Penicillamine | -COOH, -SH, -NH₂ | Tridentate (SH, NH₂, COO⁻) | Low |
Penicillamine methyl ester | -COOCH₃, -SH, -NH₂ | Tetradentate (SH, NH₂, C=O) | High |
Cysteine methyl ester | -COOCH₃, -SH, -NH₂ | Bidentate (SH, NH₂) | Moderate |
N-Acetylcysteine | -COOH, -SH, -NHAc | Bidentate (SH, COO⁻) | Low |
- Coordination Stability : Pme forms 1:1 and 1:2 complexes with lanthanides (e.g., [Ln·Pme]²⁺), with stability constants influenced by ionic radii and pH . In contrast, penicillamine’s carboxylate group limits its coordination to fewer sites, reducing complex stability in neutral media .
- Thermal Behavior : Lanthanide-Pme complexes decompose in four steps, forming Ln₂(SO₄)₃ as the final product, with activation energies inversely correlated with metal ionic radii . Similar complexes with cysteine methyl ester show less thermal stability due to simpler coordination modes .
Pharmacokinetics and Therapeutic Efficacy
Table 2: Pharmacological Comparison
- Neurological Applications: In traumatic brain injury (TBI) models, penicillamine showed superior neurological recovery despite lower brain permeability, suggesting intravascular peroxynitrite scavenging is critical .
- Peroxynitrite Scavenging: Pme is less potent than methyl chlorogenate, which exhibits twice the scavenging capacity of authentic peroxynitrite . However, Pme outperforms non-esterified thiols like glutathione in certain redox environments .
Toxicity and Limitations
- Penicillamine: Known for severe side effects, including neurotoxicity, likely due to redox interactions and metal depletion .
- This compound: Limited toxicity data, but its ester group may reduce gastrointestinal irritation compared to penicillamine’s carboxylic acid .
- Cysteine Derivatives : Lower toxicity profiles but less effective in metal chelation .
Biological Activity
Penicillamine methyl ester (Pme) is a derivative of penicillamine, a well-known chelating agent primarily used in the treatment of Wilson's disease and cystinuria. This article explores the biological activity of Pme, focusing on its mechanisms of action, pharmacokinetics, cellular effects, and potential therapeutic applications.
Overview
This compound is characterized by its ability to bind heavy metals, particularly copper, facilitating their excretion from the body. This property is crucial in treating conditions related to copper overload, such as Wilson's disease. The compound's structure includes an esterified carboxyl group, enhancing its solubility and bioavailability compared to its parent compound, penicillamine.
Target Interaction
Pme primarily targets copper ions in the body. It forms stable complexes with copper, which are then eliminated through urine. This chelation process is vital for patients suffering from Wilson's disease, where copper accumulation leads to significant organ damage.
Biochemical Pathways
The main biochemical pathway affected by Pme is copper metabolism. By binding to excess copper, Pme helps restore normal copper levels in the body. Additionally, it has been shown to inhibit macrophage activity and decrease interleukin-1 (IL-1) production, which may contribute to its anti-inflammatory effects.
Pharmacokinetics
Pme is absorbed rapidly but incompletely in the gastrointestinal tract (40-70%), with significant interindividual variability. Once absorbed, it is distributed throughout the body and primarily localized in the cytoplasm of cells where it interacts with various biomolecules.
Cellular Effects
Pme influences several cellular processes:
- T-cell Activity : It has been observed to depress T-cell activity while sparing B-cell functions, suggesting a selective immunomodulatory effect.
- Oxidative Stress Response : In vitro studies indicate that Pme can scavenge reactive oxygen species (ROS), thereby reducing oxidative stress in cells exposed to damaging agents like ionomycin and hydrogen peroxide .
Case Studies
-
Neurological Recovery Post-Injury
A study demonstrated that Pme improved neurological recovery in mice after traumatic brain injury. The compound was administered intravenously and showed a dose-dependent improvement in grip strength, indicating its potential as a neuroprotective agent during acute brain injuries . -
Spermatozoa Protection Against Oxidative Stress
Research on human spermatozoa revealed that Pme reduced ROS levels and preserved mitochondrial membrane potential and motility under oxidative stress conditions. This suggests its potential application in reproductive medicine .
Data Tables
Parameter | Value/Observation |
---|---|
Absorption Rate | 40-70% |
T-cell Activity Depression | Significant reduction observed |
Grip Score Improvement | +112% at 1.0 mg/kg; +168% at 10.0 mg/kg |
ROS Reduction in Spermatozoa | Significant decrease noted |
Q & A
Basic Research Questions
Q. What experimental design methodologies are recommended for optimizing the synthesis of Penicillamine methyl ester?
The Taguchi method is a robust statistical approach for optimizing synthesis parameters. It employs orthogonal arrays to systematically evaluate variables such as catalyst type, concentration, molar ratios, and reaction temperature. For example, potassium hydroxide (1.5 wt%) at 60°C with a 1:6 oil/methanol molar ratio achieved a 96.7% yield of methyl esters. Analysis of variance (ANOVA) and signal-to-noise (S/N) ratios help identify critical parameters, with catalyst concentration being the most influential .
Q. Which spectroscopic techniques are essential for confirming the coordination of this compound with metal ions?
Infrared (IR) spectroscopy is critical for identifying coordination sites. For lanthanide complexes, shifts in NH₂, SH, and C=O vibrational bands confirm ligand-metal binding. Elemental analysis and thermal gravimetry (TG) further validate stoichiometry (e.g., 1:1 metal-ligand ratios) and decomposition patterns .
Q. How should researchers assess the purity and structural identity of newly synthesized this compound derivatives?
Combine chromatographic (HPLC, GC-MS) and spectroscopic methods (¹H/¹³C NMR, IR). Physical properties (melting point, solubility) and elemental analysis are mandatory. For novel compounds, provide full spectral data (e.g., δH/δC values) and compare with literature. Reproducibility requires detailed experimental protocols, including solvent purity and reaction timelines .
Advanced Research Questions
Q. How do pH and ligand structural modifications influence the formation constants of this compound-lanthanide complexes?
Macroscopic protonation and formation constants (log β) are pH-dependent and determined via pH-metric titration using software like BEST. In acidic media, [Ln·Pme]²⁺ predominates, while neutral pH favors hydroxo species like [Ln·Pme·OH]⁺. Ligand substituents (e.g., methyl groups) sterically hinder cyclization, altering reaction pathways and stability. Species distribution diagrams (SPEPLOT) visualize these trends .
Q. What competing reaction pathways occur when this compound acts as a persulfide donor?
The ester releases hydropersulfide (HS⁻), which can either cyclize to form cyclic ureas or react with thiols to produce polysulfides (e.g., trisulfide 192). Competing pathways depend on linker design: bulkier substituents (e.g., 186c–d) favor thiol-mediated COS release and H₂S generation. Mechanistic studies require kinetic analysis (e.g., UV-Vis monitoring) and trapping intermediates with electrophiles .
Q. What thermal decomposition mechanisms are observed in this compound-metal complexes?
TG-DTA reveals four-stage decomposition. Initial steps involve ligand loss (150–300°C), followed by sulfate formation (500–800°C). Activation energies (ΔEa) correlate with lanthanide ionic radii, with smaller ions (e.g., Lu³⁺) requiring higher energy due to stronger metal-ligand bonds. Final residues (e.g., Ln₂(SO₄)₃) are identified via X-ray diffraction .
Q. How does this compound’s electrochemical behavior compare to other thiol-based ligands?
Cyclic voltammetry in BES buffer (pH 7.5) shows distinct redox peaks for Fe(III)-thiolate complexes. Unlike cysteine or N-acetylcysteine, the methyl ester’s electron-withdrawing group lowers reduction potentials, affecting disproportionation kinetics. Anaerobic conditions and controlled scan rates (e.g., 750 mV/s) are critical for reproducibility .
Q. Methodological Considerations
- Contradiction Analysis : Conflicting data on reaction pathways (e.g., vs. 6) may arise from pH, substituent effects, or analytical sensitivity. Replicate studies under standardized conditions (buffer, temperature) and use complementary techniques (HPLC, MS) to resolve discrepancies.
- Data Reproducibility : Document catalyst purity, solvent drying methods, and inert atmosphere protocols. Share raw data (e.g., titration curves, spectral scans) in supplementary materials .
Properties
IUPAC Name |
methyl (2S)-2-amino-3-methyl-3-sulfanylbutanoate | |
---|---|---|
Source | PubChem | |
URL | https://pubchem.ncbi.nlm.nih.gov | |
Description | Data deposited in or computed by PubChem | |
InChI |
InChI=1S/C6H13NO2S/c1-6(2,10)4(7)5(8)9-3/h4,10H,7H2,1-3H3/t4-/m0/s1 | |
Source | PubChem | |
URL | https://pubchem.ncbi.nlm.nih.gov | |
Description | Data deposited in or computed by PubChem | |
InChI Key |
FIXGPNLTDAUQQF-BYPYZUCNSA-N | |
Source | PubChem | |
URL | https://pubchem.ncbi.nlm.nih.gov | |
Description | Data deposited in or computed by PubChem | |
Canonical SMILES |
CC(C)(C(C(=O)OC)N)S | |
Source | PubChem | |
URL | https://pubchem.ncbi.nlm.nih.gov | |
Description | Data deposited in or computed by PubChem | |
Isomeric SMILES |
CC(C)([C@H](C(=O)OC)N)S | |
Source | PubChem | |
URL | https://pubchem.ncbi.nlm.nih.gov | |
Description | Data deposited in or computed by PubChem | |
Molecular Formula |
C6H13NO2S | |
Source | PubChem | |
URL | https://pubchem.ncbi.nlm.nih.gov | |
Description | Data deposited in or computed by PubChem | |
Related CAS |
34297-27-3 (hydrochloride) | |
Record name | D-Penicillamine methyl ester | |
Source | ChemIDplus | |
URL | https://pubchem.ncbi.nlm.nih.gov/substance/?source=chemidplus&sourceid=0029913835 | |
Description | ChemIDplus is a free, web search system that provides access to the structure and nomenclature authority files used for the identification of chemical substances cited in National Library of Medicine (NLM) databases, including the TOXNET system. | |
Molecular Weight |
163.24 g/mol | |
Source | PubChem | |
URL | https://pubchem.ncbi.nlm.nih.gov | |
Description | Data deposited in or computed by PubChem | |
CAS No. |
29913-83-5 | |
Record name | D-Penicillamine methyl ester | |
Source | ChemIDplus | |
URL | https://pubchem.ncbi.nlm.nih.gov/substance/?source=chemidplus&sourceid=0029913835 | |
Description | ChemIDplus is a free, web search system that provides access to the structure and nomenclature authority files used for the identification of chemical substances cited in National Library of Medicine (NLM) databases, including the TOXNET system. | |
Retrosynthesis Analysis
AI-Powered Synthesis Planning: Our tool employs the Template_relevance Pistachio, Template_relevance Bkms_metabolic, Template_relevance Pistachio_ringbreaker, Template_relevance Reaxys, Template_relevance Reaxys_biocatalysis model, leveraging a vast database of chemical reactions to predict feasible synthetic routes.
One-Step Synthesis Focus: Specifically designed for one-step synthesis, it provides concise and direct routes for your target compounds, streamlining the synthesis process.
Accurate Predictions: Utilizing the extensive PISTACHIO, BKMS_METABOLIC, PISTACHIO_RINGBREAKER, REAXYS, REAXYS_BIOCATALYSIS database, our tool offers high-accuracy predictions, reflecting the latest in chemical research and data.
Strategy Settings
Precursor scoring | Relevance Heuristic |
---|---|
Min. plausibility | 0.01 |
Model | Template_relevance |
Template Set | Pistachio/Bkms_metabolic/Pistachio_ringbreaker/Reaxys/Reaxys_biocatalysis |
Top-N result to add to graph | 6 |
Feasible Synthetic Routes
Disclaimer and Information on In-Vitro Research Products
Please be aware that all articles and product information presented on BenchChem are intended solely for informational purposes. The products available for purchase on BenchChem are specifically designed for in-vitro studies, which are conducted outside of living organisms. In-vitro studies, derived from the Latin term "in glass," involve experiments performed in controlled laboratory settings using cells or tissues. It is important to note that these products are not categorized as medicines or drugs, and they have not received approval from the FDA for the prevention, treatment, or cure of any medical condition, ailment, or disease. We must emphasize that any form of bodily introduction of these products into humans or animals is strictly prohibited by law. It is essential to adhere to these guidelines to ensure compliance with legal and ethical standards in research and experimentation.