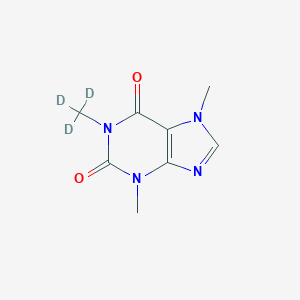
Caffeine-D3
Overview
Description
Caffeine-d3, also known as 1,3,7-trimethylxanthine-d3, is a deuterated form of caffeine. It is an isotopically labeled compound where three hydrogen atoms are replaced with deuterium. This compound is primarily used as an internal standard in mass spectrometry for the quantification of caffeine due to its similar chemical properties but distinct mass .
Preparation Methods
Synthetic Routes and Reaction Conditions
The synthesis of Caffeine-d3 involves the incorporation of deuterium into the caffeine molecule. One common method is the catalytic exchange of hydrogen atoms with deuterium in the presence of a deuterium source such as deuterium gas (D2) or deuterated solvents like deuterium oxide (D2O). The reaction typically requires a catalyst such as palladium on carbon (Pd/C) and is carried out under controlled temperature and pressure conditions .
Industrial Production Methods
Industrial production of this compound follows similar principles but on a larger scale. The process involves the use of high-pressure reactors and continuous flow systems to ensure efficient and consistent deuterium incorporation. The final product is purified using techniques such as crystallization and chromatography to achieve high purity levels required for analytical standards .
Chemical Reactions Analysis
Types of Reactions
Caffeine-d3 undergoes various chemical reactions similar to non-deuterated caffeine. These include:
Oxidation: this compound can be oxidized to form theobromine-d3 and other related compounds.
Reduction: Reduction reactions can convert this compound to dihydrothis compound.
Substitution: Nucleophilic substitution reactions can replace functional groups on the this compound molecule.
Common Reagents and Conditions
Oxidation: Common oxidizing agents include potassium permanganate (KMnO4) and hydrogen peroxide (H2O2).
Reduction: Reducing agents such as lithium aluminum hydride (LiAlH4) and sodium borohydride (NaBH4) are used.
Substitution: Reagents like alkyl halides and nucleophiles are employed under basic or acidic conditions.
Major Products Formed
The major products formed from these reactions include theobromine-d3, dihydrothis compound, and various substituted caffeine derivatives .
Scientific Research Applications
Caffeine-d3 is widely used in scientific research due to its isotopic labeling. Some key applications include:
Mechanism of Action
Caffeine-d3 exerts its effects by antagonizing adenosine receptors, particularly the A2A receptors in the brain. This antagonism leads to increased release of neurotransmitters such as dopamine and norepinephrine, resulting in enhanced alertness and wakefulness. The deuterium labeling does not significantly alter the mechanism of action compared to non-deuterated caffeine .
Comparison with Similar Compounds
Similar Compounds
Caffeine: The non-deuterated form, widely consumed as a stimulant.
Theobromine: A related compound found in chocolate, with milder stimulant effects.
Theophylline: Used in medicine for respiratory diseases due to its bronchodilator properties
Uniqueness of Caffeine-d3
Caffeine-d3 is unique due to its isotopic labeling, which makes it an ideal internal standard for analytical methods. This labeling allows for precise quantification and tracing in complex matrices, providing an advantage over non-deuterated compounds .
Biological Activity
Caffeine-D3, a labeled form of caffeine, is utilized in various research studies to explore its biological activity, particularly its effects on dopamine receptors and overall neuropharmacology. This article delves into the biological mechanisms of this compound, highlighting its interaction with dopamine D2/D3 receptors, potential psychotropic effects, and implications for health.
Caffeine acts primarily as an adenosine receptor antagonist , influencing several neurotransmitter systems:
- Dopamine Modulation : Caffeine enhances dopamine signaling by antagonizing adenosine A2A receptors (A2AR), leading to increased availability of D2/D3 receptors in the striatum. Studies have shown that a single dose of 300 mg of caffeine significantly increases D2/D3 receptor availability in the putamen and ventral striatum, correlating with heightened alertness levels among participants .
- Neurotransmitter Release : Caffeine has been observed to induce the release of dopamine and glutamate in specific brain regions, such as the nucleus accumbens. This effect is particularly pronounced in individuals with a predisposition to psychosis when caffeine is consumed in high quantities .
- Pharmacokinetics : Upon oral administration, caffeine is rapidly absorbed within 45 minutes, reaching peak plasma concentrations around 30 minutes post-consumption. Its half-life ranges from 3 to 5 hours, and it crosses the blood-brain barrier efficiently .
Case Studies
- Caffeine-Induced Psychosis : A notable case involved a 51-year-old female who consumed excessive amounts of caffeine (up to eight energy drinks daily) and experienced acute psychotic symptoms. Following cessation of caffeine intake, her symptoms resolved within five days, underscoring the potential psychiatric consequences of high caffeine consumption .
- Dopaminergic Effects : Research utilizing positron emission tomography (PET) demonstrated that caffeine administration resulted in increased D2/D3 receptor availability without elevating dopamine release itself. This suggests that caffeine may enhance dopaminergic activity by modulating receptor affinity rather than increasing synaptic dopamine levels .
Table 1: Effects of Caffeine on Dopamine Receptor Availability
Measurement Area | Placebo (Mean ± SD) | Caffeine (Mean ± SD) | p-value |
---|---|---|---|
Putamen | 2.84 ± 0.37 | 2.97 ± 0.35 | 0.05 |
Ventral Striatum | 2.69 ± 0.31 | 2.84 ± 0.39 | 0.05 |
Caudate | Not significant | Not significant | N/A |
This table summarizes findings from a study measuring the availability of D2/D3 receptors before and after caffeine administration, indicating statistically significant increases in receptor availability in specific brain regions .
Implications for Health
Caffeine's biological activity extends beyond mere wakefulness promotion; it also has implications for various health conditions:
- Cognitive Function : Regular caffeine consumption has been linked to protective effects against neurodegenerative diseases like Alzheimer's by modulating amyloid beta processing through adenosine receptor interactions .
- Bone Health : Recent studies have examined the relationship between caffeine intake and bone mineral density (BMD), indicating potential negative impacts on BMD in populations with inadequate calcium intake .
- Metabolic Effects : Caffeine influences metabolic pathways by enhancing the activity of cytochrome P450 enzymes involved in its own metabolism, which can lead to variations in individual responses to caffeine based on genetic factors .
Q & A
Basic Research Questions
Q. How is Caffeine-D3 synthesized for isotopic labeling studies, and what analytical methods validate its purity?
- Methodological Answer : Synthesis typically involves deuterium substitution at specific positions (e.g., methyl groups) via catalytic exchange or chemical reduction. Validation requires nuclear magnetic resonance (NMR) spectroscopy (e.g., <sup>2</sup>H-NMR) and mass spectrometry (MS) to confirm isotopic enrichment (>98% purity) and structural integrity. Liquid chromatography (LC-MS) is used to rule out impurities .
Q. What are the primary applications of this compound in pharmacokinetic studies?
- Methodological Answer : this compound serves as an internal standard in LC-MS/MS assays to quantify unlabeled caffeine in biological matrices (e.g., plasma, urine). Researchers use it to correct for matrix effects and ionization variability. Protocols involve spiking known concentrations into samples before extraction, followed by calibration curve analysis .
Q. How do researchers ensure the stability of this compound in experimental storage conditions?
- Methodological Answer : Stability testing includes accelerated degradation studies under varying temperatures (4°C, -20°C) and pH levels. High-performance liquid chromatography (HPLC) monitors deuterium loss over time. Best practices recommend aliquoting and storing in inert atmospheres (argon) to minimize isotopic exchange .
Advanced Research Questions
Q. What experimental designs address conflicting data on this compound’s metabolic half-life across in vitro and in vivo models? **
- Methodological Answer : Contradictions arise from differences in cytochrome P450 (CYP1A2) activity across species. A hybrid design combines:
- In vitro : Microsomal assays with human/rodent liver enzymes.
- In vivo : Cross-over trials in transgenic mice expressing human CYP1A2.
Data normalization uses body surface area scaling and allometric modeling .
Q. How can isotopic effects of deuterium in this compound bias metabolic pathway analyses?
- Methodological Answer : Deuterium’s kinetic isotope effect (KIE) alters reaction rates, particularly in demethylation steps. Researchers mitigate this by:
- Comparing metabolic profiles of this compound vs. non-deuterated analogs via tandem MS.
- Applying computational models (e.g., density functional theory) to predict KIE magnitudes.
- Validating findings with <sup>13</sup>C-labeled caffeine as a control .
Q. What statistical frameworks reconcile discrepancies in this compound’s tissue distribution data across studies?
- Methodological Answer : Meta-analytic approaches aggregate data from multiple studies, adjusting for covariates like dosage, administration route, and detection limits. Bayesian hierarchical models account for inter-study heterogeneity, while sensitivity analyses identify outlier datasets. Open-source tools (e.g., R package “metafor”) facilitate reproducibility .
Q. How do researchers optimize this compound protocols for dual isotope tracing (e.g., combined <sup>2</sup>H/<sup>13</sup>C labeling)?
- Methodological Answer : Dual labeling requires orthogonal detection methods (e.g., NMR for <sup>2</sup>H, MS for <sup>13</sup>C). Experimental optimization includes:
- Synthesis : Stepwise deuterium incorporation followed by <sup>13</sup>C enrichment at carboxyl groups.
- Analysis : Parallel reaction monitoring (PRM) in MS to distinguish isotopic clusters.
Validation via spike-recovery experiments in complex matrices .
Q. Data Analysis & Interpretation
Q. What criteria distinguish high-quality this compound studies in systematic reviews?
- Methodological Answer : Use the FINER framework (Feasible, Interesting, Novel, Ethical, Relevant) to evaluate studies. Key markers include:
- Transparency : Full disclosure of synthetic routes, purity data, and MS/MS parameters.
- Reproducibility : Availability of raw spectral data in repositories (e.g., Zenodo).
- Ethics : Compliance with isotopic waste disposal guidelines .
Q. How are machine learning models applied to predict this compound’s environmental persistence?
- Methodological Answer : Quantitative structure-activity relationship (QSAR) models train on datasets of deuterated compounds’ biodegradation rates. Features include logP, deuterium substitution sites, and molecular polarity. Validation uses leave-one-out cross-validation and comparison with experimental half-lives in soil/water systems .
Q. Interdisciplinary Research Considerations
Q. What ethical and technical challenges arise when using this compound in human neuropharmacology trials?
- Methodological Answer : Challenges include:
- Ethical : Justifying deuterium exposure risks in vulnerable populations (e.g., pregnant individuals).
- Technical : Ensuring isotopic purity meets FDA guidelines for internal standards.
Solutions involve preclinical toxicokinetic studies and third-party certification of synthesis labs .
Properties
IUPAC Name |
3,7-dimethyl-1-(trideuteriomethyl)purine-2,6-dione | |
---|---|---|
Source | PubChem | |
URL | https://pubchem.ncbi.nlm.nih.gov | |
Description | Data deposited in or computed by PubChem | |
InChI |
InChI=1S/C8H10N4O2/c1-10-4-9-6-5(10)7(13)12(3)8(14)11(6)2/h4H,1-3H3/i3D3 | |
Source | PubChem | |
URL | https://pubchem.ncbi.nlm.nih.gov | |
Description | Data deposited in or computed by PubChem | |
InChI Key |
RYYVLZVUVIJVGH-HPRDVNIFSA-N | |
Source | PubChem | |
URL | https://pubchem.ncbi.nlm.nih.gov | |
Description | Data deposited in or computed by PubChem | |
Canonical SMILES |
CN1C=NC2=C1C(=O)N(C(=O)N2C)C | |
Source | PubChem | |
URL | https://pubchem.ncbi.nlm.nih.gov | |
Description | Data deposited in or computed by PubChem | |
Isomeric SMILES |
[2H]C([2H])([2H])N1C(=O)C2=C(N=CN2C)N(C1=O)C | |
Source | PubChem | |
URL | https://pubchem.ncbi.nlm.nih.gov | |
Description | Data deposited in or computed by PubChem | |
Molecular Formula |
C8H10N4O2 | |
Source | PubChem | |
URL | https://pubchem.ncbi.nlm.nih.gov | |
Description | Data deposited in or computed by PubChem | |
DSSTOX Substance ID |
DTXSID00514758 | |
Record name | 3,7-Dimethyl-1-(trideuteriomethyl)purine-2,6-dione | |
Source | EPA DSSTox | |
URL | https://comptox.epa.gov/dashboard/DTXSID00514758 | |
Description | DSSTox provides a high quality public chemistry resource for supporting improved predictive toxicology. | |
Molecular Weight |
197.21 g/mol | |
Source | PubChem | |
URL | https://pubchem.ncbi.nlm.nih.gov | |
Description | Data deposited in or computed by PubChem | |
CAS No. |
26351-03-1 | |
Record name | 3,7-Dimethyl-1-(trideuteriomethyl)purine-2,6-dione | |
Source | EPA DSSTox | |
URL | https://comptox.epa.gov/dashboard/DTXSID00514758 | |
Description | DSSTox provides a high quality public chemistry resource for supporting improved predictive toxicology. | |
Retrosynthesis Analysis
AI-Powered Synthesis Planning: Our tool employs the Template_relevance Pistachio, Template_relevance Bkms_metabolic, Template_relevance Pistachio_ringbreaker, Template_relevance Reaxys, Template_relevance Reaxys_biocatalysis model, leveraging a vast database of chemical reactions to predict feasible synthetic routes.
One-Step Synthesis Focus: Specifically designed for one-step synthesis, it provides concise and direct routes for your target compounds, streamlining the synthesis process.
Accurate Predictions: Utilizing the extensive PISTACHIO, BKMS_METABOLIC, PISTACHIO_RINGBREAKER, REAXYS, REAXYS_BIOCATALYSIS database, our tool offers high-accuracy predictions, reflecting the latest in chemical research and data.
Strategy Settings
Precursor scoring | Relevance Heuristic |
---|---|
Min. plausibility | 0.01 |
Model | Template_relevance |
Template Set | Pistachio/Bkms_metabolic/Pistachio_ringbreaker/Reaxys/Reaxys_biocatalysis |
Top-N result to add to graph | 6 |
Feasible Synthetic Routes
Disclaimer and Information on In-Vitro Research Products
Please be aware that all articles and product information presented on BenchChem are intended solely for informational purposes. The products available for purchase on BenchChem are specifically designed for in-vitro studies, which are conducted outside of living organisms. In-vitro studies, derived from the Latin term "in glass," involve experiments performed in controlled laboratory settings using cells or tissues. It is important to note that these products are not categorized as medicines or drugs, and they have not received approval from the FDA for the prevention, treatment, or cure of any medical condition, ailment, or disease. We must emphasize that any form of bodily introduction of these products into humans or animals is strictly prohibited by law. It is essential to adhere to these guidelines to ensure compliance with legal and ethical standards in research and experimentation.