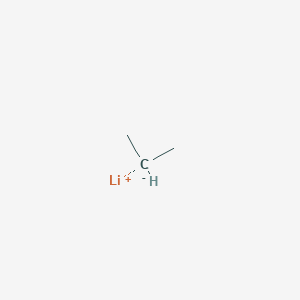
Isopropyllithium
Overview
Description
Isopropyllithium, also known as 2-propyllithium or lithium propan-2-ide, is an organolithium compound with the chemical formula C3H7Li. It is a colorless liquid that exhibits strong reducibility and alkalinity. Due to its high reactivity, this compound must be handled under an inert atmosphere, such as argon, to prevent reactions with moisture and oxygen in the air .
Preparation Methods
Synthetic Routes and Reaction Conditions: Isopropyllithium is typically synthesized by the reaction of ethanyllithium with isopropanol. The reaction proceeds as follows:
C2H5Li+(CH3)2CHOH→C3H7Li+C2H5OH
This reaction is carried out under an inert atmosphere to prevent the highly reactive this compound from reacting with moisture or oxygen .
Industrial Production Methods: In industrial settings, this compound is often produced in a solution, such as in pentane or hexane, to stabilize the compound and facilitate its handling. The concentration of this compound in these solutions is typically around 0.7 M .
Chemical Reactions Analysis
Types of Reactions: Isopropyllithium undergoes various types of chemical reactions, including:
Addition Reactions: It adds to carbonyl compounds to form alcohols.
Substitution Reactions: It can substitute halogens in organic halides.
Deprotonation Reactions: It acts as a strong base to deprotonate weak acids.
Common Reagents and Conditions:
Carbonyl Compounds: this compound adds to aldehydes and ketones to form secondary and tertiary alcohols, respectively.
Organic Halides: It can substitute halogens in organic halides under anhydrous conditions.
Major Products:
Alcohols: The addition of this compound to carbonyl compounds results in the formation of alcohols.
Substituted Organic Compounds: Substitution reactions with organic halides yield substituted organic compounds.
Scientific Research Applications
Isopropyllithium is widely used in scientific research due to its versatility and reactivity. Some of its applications include:
Organic Synthesis: It is used as a strong base, reducing agent, and catalyst in various organic reactions, such as aldol reactions and rearrangement reactions.
Drug Synthesis: It is employed in the synthesis of certain pharmaceuticals.
Material Science: It is used in the production of lithium-containing organic compounds and metal-organic frameworks.
Polymer Chemistry: It is utilized in the synthesis of synthetic resins and rubber.
Mechanism of Action
Isopropyllithium exerts its effects primarily through nucleophilic addition and substitution reactions. The mechanism involves the formation of a carbanion, which acts as a nucleophile to attack electrophilic centers in target molecules. The reactivity of this compound is influenced by its aggregation state, which can vary depending on the solvent and concentration .
Comparison with Similar Compounds
Isopropyllithium is similar to other organolithium compounds, such as:
- Methyllithium (CH3Li)
- Ethyllithium (C2H5Li)
- n-Butyllithium (C4H9Li)
Uniqueness:
- Reactivity: this compound is more reactive than isopropylmagnesium bromide in certain addition reactions .
- Aggregation State: It exhibits different aggregation states (tetrameric and hexameric) depending on the solvent and concentration, which can influence its reactivity .
This compound’s unique properties make it a valuable reagent in various chemical syntheses and industrial applications.
Properties
IUPAC Name |
lithium;propane | |
---|---|---|
Source | PubChem | |
URL | https://pubchem.ncbi.nlm.nih.gov | |
Description | Data deposited in or computed by PubChem | |
InChI |
InChI=1S/C3H7.Li/c1-3-2;/h3H,1-2H3;/q-1;+1 | |
Source | PubChem | |
URL | https://pubchem.ncbi.nlm.nih.gov | |
Description | Data deposited in or computed by PubChem | |
InChI Key |
SZAVVKVUMPLRRS-UHFFFAOYSA-N | |
Source | PubChem | |
URL | https://pubchem.ncbi.nlm.nih.gov | |
Description | Data deposited in or computed by PubChem | |
Canonical SMILES |
[Li+].C[CH-]C | |
Source | PubChem | |
URL | https://pubchem.ncbi.nlm.nih.gov | |
Description | Data deposited in or computed by PubChem | |
Molecular Formula |
C3H7Li | |
Source | PubChem | |
URL | https://pubchem.ncbi.nlm.nih.gov | |
Description | Data deposited in or computed by PubChem | |
DSSTOX Substance ID |
DTXSID40940390 | |
Record name | Lithium propan-2-ide | |
Source | EPA DSSTox | |
URL | https://comptox.epa.gov/dashboard/DTXSID40940390 | |
Description | DSSTox provides a high quality public chemistry resource for supporting improved predictive toxicology. | |
Molecular Weight |
50.1 g/mol | |
Source | PubChem | |
URL | https://pubchem.ncbi.nlm.nih.gov | |
Description | Data deposited in or computed by PubChem | |
CAS No. |
1888-75-1 | |
Record name | Isopropyllithium | |
Source | ChemIDplus | |
URL | https://pubchem.ncbi.nlm.nih.gov/substance/?source=chemidplus&sourceid=0001888751 | |
Description | ChemIDplus is a free, web search system that provides access to the structure and nomenclature authority files used for the identification of chemical substances cited in National Library of Medicine (NLM) databases, including the TOXNET system. | |
Record name | Lithium propan-2-ide | |
Source | EPA DSSTox | |
URL | https://comptox.epa.gov/dashboard/DTXSID40940390 | |
Description | DSSTox provides a high quality public chemistry resource for supporting improved predictive toxicology. | |
Record name | Isopropyllithium | |
Source | European Chemicals Agency (ECHA) | |
URL | https://echa.europa.eu/substance-information/-/substanceinfo/100.015.966 | |
Description | The European Chemicals Agency (ECHA) is an agency of the European Union which is the driving force among regulatory authorities in implementing the EU's groundbreaking chemicals legislation for the benefit of human health and the environment as well as for innovation and competitiveness. | |
Explanation | Use of the information, documents and data from the ECHA website is subject to the terms and conditions of this Legal Notice, and subject to other binding limitations provided for under applicable law, the information, documents and data made available on the ECHA website may be reproduced, distributed and/or used, totally or in part, for non-commercial purposes provided that ECHA is acknowledged as the source: "Source: European Chemicals Agency, http://echa.europa.eu/". Such acknowledgement must be included in each copy of the material. ECHA permits and encourages organisations and individuals to create links to the ECHA website under the following cumulative conditions: Links can only be made to webpages that provide a link to the Legal Notice page. | |
Retrosynthesis Analysis
AI-Powered Synthesis Planning: Our tool employs the Template_relevance Pistachio, Template_relevance Bkms_metabolic, Template_relevance Pistachio_ringbreaker, Template_relevance Reaxys, Template_relevance Reaxys_biocatalysis model, leveraging a vast database of chemical reactions to predict feasible synthetic routes.
One-Step Synthesis Focus: Specifically designed for one-step synthesis, it provides concise and direct routes for your target compounds, streamlining the synthesis process.
Accurate Predictions: Utilizing the extensive PISTACHIO, BKMS_METABOLIC, PISTACHIO_RINGBREAKER, REAXYS, REAXYS_BIOCATALYSIS database, our tool offers high-accuracy predictions, reflecting the latest in chemical research and data.
Strategy Settings
Precursor scoring | Relevance Heuristic |
---|---|
Min. plausibility | 0.01 |
Model | Template_relevance |
Template Set | Pistachio/Bkms_metabolic/Pistachio_ringbreaker/Reaxys/Reaxys_biocatalysis |
Top-N result to add to graph | 6 |
Feasible Synthetic Routes
Q1: What is the molecular formula and weight of isopropyllithium?
A1: this compound has the molecular formula C3H7Li and a molecular weight of 50.08 g/mol.
Q2: What spectroscopic techniques are useful for characterizing this compound?
A2: Nuclear magnetic resonance (NMR) spectroscopy, particularly 7Li NMR and 1H NMR, is valuable for studying the structure and dynamics of this compound. 7Li NMR provides insights into the aggregation state and coordination environment of lithium, while 1H NMR can be used to study the isopropyl group and any coordinated ligands. [, ] Additionally, 13C NMR can provide information on the structure of this compound adducts with dienes. []
Q3: Can you describe the reactivity of this compound towards α-substituted styrenes?
A3: When reacted with α-substituted styrenes in diethyl ether, this compound adds to the vinyl group. The stability of the resulting cyclopropylcarbinyllithium species formed during the reaction is influenced by the substituents on the styrene. []
Q4: How does this compound react with simple olefins like ethylene and propene?
A4: The reaction of this compound with simple olefins like ethylene and propene leads to addition products. [] The specific reaction conditions and the structure of the olefin can impact the reaction pathway and the final product distribution.
Q5: How is this compound used in the synthesis of (R)-2-butanol derivatives?
A5: this compound acts as a nucleophile in the ring-opening reaction of optically pure (R)-(+)-1,2-epoxybutane, leading to the formation of new (R)-2-butanol derivatives. [] This reaction offers a stereoselective route to access specific isomers of these valuable chiral building blocks.
Q6: What is the role of this compound in the synthesis of tetraorganyldiboranes(4)?
A6: this compound serves as an alkylating agent in the synthesis of tetraorganyldiboranes(4) from B2(OCH3)4. Unlike reactions with tert-butyllithium, the reaction with this compound proceeds directly to the tetraalkylated product without the isolation of intermediate alkoxydiboranes. []
Q7: How does this compound behave in the presence of chiral diamines like sparteine?
A7: this compound forms complexes with chiral diamines like sparteine, which are used for enantioselective deprotonation reactions. [] The structure and reactivity of these complexes are influenced by the specific diamine used and the reaction conditions. For example, an unsymmetrically complexed this compound/sparteine/Et2O dimer has been characterized and its reactivity studied. []
Q8: What is the significance of this compound in enantioselective lithiation reactions of N-Boc-pyrrolidine?
A8: this compound, in conjunction with chiral diamines like sparteine, can achieve the enantioselective lithiation of N-Boc-pyrrolidine. [] This reaction provides access to enantioenriched pyrrolidine derivatives, which are important building blocks for various pharmaceuticals and natural products. The enantioselectivity is influenced by the structure of the chiral diamine, with steric hindrance playing a significant role. []
Q9: How does this compound interact with chiral lithium amides?
A9: this compound can form mixed aggregates with chiral lithium amides, such as those derived from N-ethyl-O-triisopropylsilyl valinol. [] These mixed aggregates typically adopt ladder-like structures both in the solid state and in toluene solution. [] The formation and structure of these aggregates are relevant to understanding the reactivity and selectivity of this compound in the presence of chiral ligands.
Q10: Have computational methods been used to study this compound?
A10: Yes, computational chemistry has been employed to study the structure, bonding, and reactivity of this compound. For instance, density functional theory (DFT) calculations have provided insights into the aggregation behavior and solvation of this compound in different solvents. [] Furthermore, computational studies have been utilized to investigate the mechanisms of reactions involving this compound, including enantioselective deprotonation reactions. [, ] These calculations help to rationalize experimental observations and to predict the stereochemical outcome of reactions.
Disclaimer and Information on In-Vitro Research Products
Please be aware that all articles and product information presented on BenchChem are intended solely for informational purposes. The products available for purchase on BenchChem are specifically designed for in-vitro studies, which are conducted outside of living organisms. In-vitro studies, derived from the Latin term "in glass," involve experiments performed in controlled laboratory settings using cells or tissues. It is important to note that these products are not categorized as medicines or drugs, and they have not received approval from the FDA for the prevention, treatment, or cure of any medical condition, ailment, or disease. We must emphasize that any form of bodily introduction of these products into humans or animals is strictly prohibited by law. It is essential to adhere to these guidelines to ensure compliance with legal and ethical standards in research and experimentation.