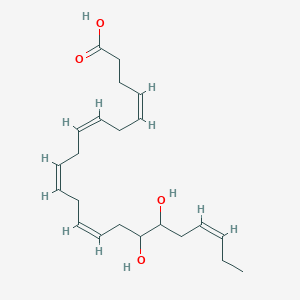
(±)16(17)-DiHDPA
Overview
Description
(±)16(17)-DiHDPA is a polyunsaturated fatty acid with multiple double bonds and hydroxyl groups. This compound is of significant interest due to its potential biological activities and applications in various fields, including chemistry, biology, and medicine.
Mechanism of Action
Target of Action
16,17-DiHDPE, also known as (+/-)-16,17-dihydroxy-4Z,7Z,10Z,13Z,19Z-docosapentaenoic acid, primarily targets the soluble epoxide hydrolase (sEH) enzyme . This enzyme plays a crucial role in the metabolism of fatty acids, particularly polyunsaturated fatty acids (PUFAs), and is involved in various biological functions .
Mode of Action
16,17-DiHDPE is produced from the action of the cytochrome P450 epoxygenase on docosahexaenoic acid (DHA) . The compound interacts with its target, the sEH enzyme, which hydrolyzes the epoxy fatty acid metabolites of linoleic acid (EpOMEs) to dihydroxyoctadecenoic acids (DiHOMEs) . This interaction results in changes in the concentrations of these metabolites .
Biochemical Pathways
The production of 16,17-DiHDPE involves the cytochrome P450 (CYP450) pathway . In this pathway, the CYP450-formed epoxy fatty acid metabolites of linoleic acid (EpOMEs) are hydrolyzed by the sEH enzyme to form DiHOMEs . These DiHOMEs are considered cardioprotective at low concentrations but have been implicated as vascular permeability and cytotoxic agents at higher levels .
Pharmacokinetics
It is known that the compound is a metabolite of dha, which is an essential fatty acid and the most abundant ω-3 fatty acid in neural tissues .
Result of Action
16,17-DiHDPE has been shown to inhibit VEGF-induced angiogenesis and reduce cancer cell metastasis in mice . It also plays a role in driving pathologic inflammation while impairing key innate immune cell function in burn injury . Specifically, it facilitates the maturation and activation of stimulated neutrophils, while impeding monocyte and macrophage functionality and cytokine generation .
Action Environment
The action of 16,17-DiHDPE can be influenced by various environmental factors. For instance, the fatty acid composition in the Western diet, which has shifted from saturated to polyunsaturated fatty acids (PUFAs), specifically omega-6 linoleic acid (LA, 18:2), can affect the production and action of 16,17-DiHDPE . Additionally, the administration of certain compounds, such as 1-trifluoromethoxyphenyl-3-(1-propionylpiperidin-4-yl) urea (TPPU), a sEH inhibitor, can influence the concentrations of 16,17-DiHDPE .
Biochemical Analysis
Biochemical Properties
16,17-Dihydroxy-4Z,7Z,10Z,13Z,19Z-docosapentaenoic acid interacts with various enzymes, proteins, and other biomolecules. It is a product of the cytochrome P450 (CYP450)–formed epoxy fatty acid metabolites of DHA, which are hydrolyzed by the soluble epoxide hydrolase enzyme (sEH) to dihydroxydocosapentaenoic acids . This compound has been associated with various biological functions, including cardioprotection at low concentrations .
Cellular Effects
The effects of 16,17-Dihydroxy-4Z,7Z,10Z,13Z,19Z-docosapentaenoic acid on various types of cells and cellular processes are significant. It has been found to facilitate the maturation and activation of stimulated neutrophils, while impeding monocyte and macrophage functionality and cytokine generation . This compound influences cell function, including impacts on cell signaling pathways, gene expression, and cellular metabolism .
Molecular Mechanism
The mechanism of action of 16,17-Dihydroxy-4Z,7Z,10Z,13Z,19Z-docosapentaenoic acid involves its interactions with biomolecules at the molecular level. It exerts its effects through binding interactions with biomolecules, enzyme inhibition or activation, and changes in gene expression .
Metabolic Pathways
16,17-Dihydroxy-4Z,7Z,10Z,13Z,19Z-docosapentaenoic acid is involved in the metabolic pathways of DHA. It interacts with enzymes such as cytochrome P450 and soluble epoxide hydrolase, and may affect metabolic flux or metabolite levels .
Preparation Methods
Synthetic Routes and Reaction Conditions
The synthesis of (±)16(17)-DiHDPA typically involves multiple steps, including the introduction of double bonds and hydroxyl groups at specific positions. One common method involves the use of palladium-catalyzed cross-coupling reactions to form the carbon-carbon double bonds, followed by selective hydroxylation to introduce the hydroxyl groups. The reaction conditions often require precise control of temperature, pressure, and the use of specific catalysts to achieve the desired product.
Industrial Production Methods
Industrial production of this compound may involve large-scale synthesis using similar methods as in the laboratory but optimized for higher yields and efficiency. This can include the use of continuous flow reactors, advanced purification techniques, and automation to ensure consistent quality and scalability.
Chemical Reactions Analysis
Types of Reactions
(±)16(17)-DiHDPA can undergo various chemical reactions, including:
Oxidation: The hydroxyl groups can be oxidized to form ketones or aldehydes.
Reduction: The double bonds can be reduced to form saturated fatty acids.
Substitution: The hydroxyl groups can be substituted with other functional groups, such as halogens or amines.
Common Reagents and Conditions
Oxidation: Common oxidizing agents include potassium permanganate (KMnO4) and chromium trioxide (CrO3).
Reduction: Hydrogen gas (H2) in the presence of a palladium catalyst (Pd/C) is often used for the reduction of double bonds.
Substitution: Reagents such as thionyl chloride (SOCl2) for halogenation or ammonia (NH3) for amination are commonly used.
Major Products
Oxidation: Formation of ketones or aldehydes.
Reduction: Formation of saturated fatty acids.
Substitution: Formation of halogenated or aminated derivatives.
Scientific Research Applications
(±)16(17)-DiHDPA has a wide range of applications in scientific research:
- Medicine
Chemistry: Used as a precursor for the synthesis of more complex molecules and as a model compound for studying reaction mechanisms.
Biology: Investigated for its role in cellular signaling and metabolism.
Properties
IUPAC Name |
(4Z,7Z,10Z,13Z,19Z)-16,17-dihydroxydocosa-4,7,10,13,19-pentaenoic acid | |
---|---|---|
Source | PubChem | |
URL | https://pubchem.ncbi.nlm.nih.gov | |
Description | Data deposited in or computed by PubChem | |
InChI |
InChI=1S/C22H34O4/c1-2-3-14-17-20(23)21(24)18-15-12-10-8-6-4-5-7-9-11-13-16-19-22(25)26/h3,5-8,11-15,20-21,23-24H,2,4,9-10,16-19H2,1H3,(H,25,26)/b7-5-,8-6-,13-11-,14-3-,15-12- | |
Source | PubChem | |
URL | https://pubchem.ncbi.nlm.nih.gov | |
Description | Data deposited in or computed by PubChem | |
InChI Key |
YXQCSWUATWXVGK-ZYADFMMDSA-N | |
Source | PubChem | |
URL | https://pubchem.ncbi.nlm.nih.gov | |
Description | Data deposited in or computed by PubChem | |
Canonical SMILES |
CCC=CCC(C(CC=CCC=CCC=CCC=CCCC(=O)O)O)O | |
Source | PubChem | |
URL | https://pubchem.ncbi.nlm.nih.gov | |
Description | Data deposited in or computed by PubChem | |
Isomeric SMILES |
CC/C=C\CC(C(C/C=C\C/C=C\C/C=C\C/C=C\CCC(=O)O)O)O | |
Source | PubChem | |
URL | https://pubchem.ncbi.nlm.nih.gov | |
Description | Data deposited in or computed by PubChem | |
Molecular Formula |
C22H34O4 | |
Source | PubChem | |
URL | https://pubchem.ncbi.nlm.nih.gov | |
Description | Data deposited in or computed by PubChem | |
Molecular Weight |
362.5 g/mol | |
Source | PubChem | |
URL | https://pubchem.ncbi.nlm.nih.gov | |
Description | Data deposited in or computed by PubChem | |
Retrosynthesis Analysis
AI-Powered Synthesis Planning: Our tool employs the Template_relevance Pistachio, Template_relevance Bkms_metabolic, Template_relevance Pistachio_ringbreaker, Template_relevance Reaxys, Template_relevance Reaxys_biocatalysis model, leveraging a vast database of chemical reactions to predict feasible synthetic routes.
One-Step Synthesis Focus: Specifically designed for one-step synthesis, it provides concise and direct routes for your target compounds, streamlining the synthesis process.
Accurate Predictions: Utilizing the extensive PISTACHIO, BKMS_METABOLIC, PISTACHIO_RINGBREAKER, REAXYS, REAXYS_BIOCATALYSIS database, our tool offers high-accuracy predictions, reflecting the latest in chemical research and data.
Strategy Settings
Precursor scoring | Relevance Heuristic |
---|---|
Min. plausibility | 0.01 |
Model | Template_relevance |
Template Set | Pistachio/Bkms_metabolic/Pistachio_ringbreaker/Reaxys/Reaxys_biocatalysis |
Top-N result to add to graph | 6 |
Feasible Synthetic Routes
A: The study found that celecoxib, while aiming to inhibit COX2 and reduce pro-inflammatory prostaglandin E2 (PGE2), led to an increase in certain oxylipins, including 16,17-DiHDPE []. This is significant because it suggests that inhibiting one pathway of arachidonic acid metabolism might lead to a compensatory increase in other pathways, potentially leading to unintended consequences. While the exact effects of elevated 16,17-DiHDPE are not fully understood, the study highlights the complex interplay within the arachidonic acid metabolic network and raises questions about the long-term effects of selectively targeting specific pathways. Further research is needed to elucidate the specific roles of 16,17-DiHDPE and other oxylipins affected by celecoxib to fully assess the implications of these findings for colorectal cancer chemoprevention strategies.
Disclaimer and Information on In-Vitro Research Products
Please be aware that all articles and product information presented on BenchChem are intended solely for informational purposes. The products available for purchase on BenchChem are specifically designed for in-vitro studies, which are conducted outside of living organisms. In-vitro studies, derived from the Latin term "in glass," involve experiments performed in controlled laboratory settings using cells or tissues. It is important to note that these products are not categorized as medicines or drugs, and they have not received approval from the FDA for the prevention, treatment, or cure of any medical condition, ailment, or disease. We must emphasize that any form of bodily introduction of these products into humans or animals is strictly prohibited by law. It is essential to adhere to these guidelines to ensure compliance with legal and ethical standards in research and experimentation.