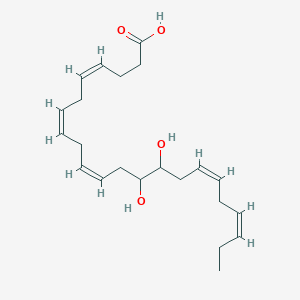
(+/-)-13,14-dihydroxy-4Z,7Z,10Z,16Z,19Z-docosapentaenoic acid
Overview
Description
The description of an organic compound usually includes its molecular formula, structure, and functional groups. The compound’s name often gives insight into its structure, as it follows the rules of IUPAC nomenclature .
Synthesis Analysis
The synthesis of an organic compound involves a series of chemical reactions, starting from readily available substances. The process often requires catalysts and specific conditions of temperature and pressure .Molecular Structure Analysis
The molecular structure of a compound is determined using various spectroscopic techniques, such as infrared (IR) spectroscopy, nuclear magnetic resonance (NMR), and X-ray crystallography .Chemical Reactions Analysis
This involves studying how the compound reacts with other substances. The type of reactions that a compound undergoes can tell us a lot about its functional groups .Physical And Chemical Properties Analysis
The physical and chemical properties of a compound include its melting and boiling points, solubility in various solvents, and its reactivity with common reagents .Scientific Research Applications
Metabolite of Docosahexaenoic Acid (DHA)
13,14-DiHDPE is a metabolite of docosahexaenoic acid (DHA), which is an omega-3 fatty acid that is a primary structural component of the human brain, cerebral cortex, skin, and retina .
Produced via Oxidation by Cytochrome P450 Epoxygenases
This compound is produced via oxidation by cytochrome P450 epoxygenases . These enzymes are involved in the metabolism of various substances in the body.
Inhibition of Angiogenesis
Angiogenesis, the formation of new blood vessels, plays a crucial role in many physiological and pathological processes. 13,14-DiHDPE is reported to inhibit angiogenesis , which could have implications in treating diseases such as cancer, where inhibiting the blood supply to a tumor can help to prevent its growth.
Tumor Growth Inhibition
13,14-DiHDPE has been reported to inhibit tumor growth . This could make it a potential therapeutic agent in the treatment of various types of cancer.
Inhibition of Metastasis
Metastasis, the spread of cancer cells from the place where they first formed to another part of the body, is a major cause of cancer death. 13,14-DiHDPE is reported to inhibit metastasis , which could have significant implications in the treatment of cancer.
Potential Role in Lipid Biochemistry
Given that 13,14-DiHDPE is a metabolite of DHA, it may play a role in lipid biochemistry . Further research is needed to fully understand this potential application.
Mechanism of Action
Target of Action
13,14-DiHDPE, also known as (4Z,7Z,10Z,16Z,19Z)-13,14-Dihydroxydocosa-4,7,10,16,19-pentaenoic acid, is a metabolite of docosahexaenoic acid (DHA). It is produced via oxidation by cytochrome P450 epoxygenases . These enzymes are the primary targets of 13,14-DiHDPE and play a crucial role in its mechanism of action.
Mode of Action
The interaction of 13,14-DiHDPE with cytochrome P450 epoxygenases results in the production of epoxygenase metabolites of DHA . These metabolites, including 13,14-DiHDPE, have been reported to inhibit angiogenesis, tumor growth, and metastasis .
Biochemical Pathways
The biochemical pathway involved in the action of 13,14-DiHDPE is the cytochrome P450 pathway . This pathway is responsible for the oxidation of DHA, leading to the production of 13,14-DiHDPE. The metabolites produced via this pathway have significant effects on various biological processes, including angiogenesis, tumor growth, and metastasis .
Pharmacokinetics
It is known that 13,14-dihdpe is a metabolite of dha and is produced via oxidation by cytochrome p450 epoxygenases .
Result of Action
The action of 13,14-DiHDPE results in the inhibition of angiogenesis, tumor growth, and metastasis . This is achieved through the production of epoxygenase metabolites of DHA, which have significant effects on these biological processes .
Safety and Hazards
Future Directions
properties
IUPAC Name |
(4Z,7Z,10Z,16Z,19Z)-13,14-dihydroxydocosa-4,7,10,16,19-pentaenoic acid | |
---|---|---|
Source | PubChem | |
URL | https://pubchem.ncbi.nlm.nih.gov | |
Description | Data deposited in or computed by PubChem | |
InChI |
InChI=1S/C22H34O4/c1-2-3-4-5-11-14-17-20(23)21(24)18-15-12-9-7-6-8-10-13-16-19-22(25)26/h3-4,6-7,10-15,20-21,23-24H,2,5,8-9,16-19H2,1H3,(H,25,26)/b4-3-,7-6-,13-10-,14-11-,15-12- | |
Source | PubChem | |
URL | https://pubchem.ncbi.nlm.nih.gov | |
Description | Data deposited in or computed by PubChem | |
InChI Key |
LINXWSBRRJSWHL-UQZHZJRSSA-N | |
Source | PubChem | |
URL | https://pubchem.ncbi.nlm.nih.gov | |
Description | Data deposited in or computed by PubChem | |
Canonical SMILES |
CCC=CCC=CCC(C(CC=CCC=CCC=CCCC(=O)O)O)O | |
Source | PubChem | |
URL | https://pubchem.ncbi.nlm.nih.gov | |
Description | Data deposited in or computed by PubChem | |
Isomeric SMILES |
CC/C=C\C/C=C\CC(C(C/C=C\C/C=C\C/C=C\CCC(=O)O)O)O | |
Source | PubChem | |
URL | https://pubchem.ncbi.nlm.nih.gov | |
Description | Data deposited in or computed by PubChem | |
Molecular Formula |
C22H34O4 | |
Source | PubChem | |
URL | https://pubchem.ncbi.nlm.nih.gov | |
Description | Data deposited in or computed by PubChem | |
Molecular Weight |
362.5 g/mol | |
Source | PubChem | |
URL | https://pubchem.ncbi.nlm.nih.gov | |
Description | Data deposited in or computed by PubChem | |
Product Name |
(+/-)-13,14-dihydroxy-4Z,7Z,10Z,16Z,19Z-docosapentaenoic acid |
Retrosynthesis Analysis
AI-Powered Synthesis Planning: Our tool employs the Template_relevance Pistachio, Template_relevance Bkms_metabolic, Template_relevance Pistachio_ringbreaker, Template_relevance Reaxys, Template_relevance Reaxys_biocatalysis model, leveraging a vast database of chemical reactions to predict feasible synthetic routes.
One-Step Synthesis Focus: Specifically designed for one-step synthesis, it provides concise and direct routes for your target compounds, streamlining the synthesis process.
Accurate Predictions: Utilizing the extensive PISTACHIO, BKMS_METABOLIC, PISTACHIO_RINGBREAKER, REAXYS, REAXYS_BIOCATALYSIS database, our tool offers high-accuracy predictions, reflecting the latest in chemical research and data.
Strategy Settings
Precursor scoring | Relevance Heuristic |
---|---|
Min. plausibility | 0.01 |
Model | Template_relevance |
Template Set | Pistachio/Bkms_metabolic/Pistachio_ringbreaker/Reaxys/Reaxys_biocatalysis |
Top-N result to add to graph | 6 |
Feasible Synthetic Routes
Disclaimer and Information on In-Vitro Research Products
Please be aware that all articles and product information presented on BenchChem are intended solely for informational purposes. The products available for purchase on BenchChem are specifically designed for in-vitro studies, which are conducted outside of living organisms. In-vitro studies, derived from the Latin term "in glass," involve experiments performed in controlled laboratory settings using cells or tissues. It is important to note that these products are not categorized as medicines or drugs, and they have not received approval from the FDA for the prevention, treatment, or cure of any medical condition, ailment, or disease. We must emphasize that any form of bodily introduction of these products into humans or animals is strictly prohibited by law. It is essential to adhere to these guidelines to ensure compliance with legal and ethical standards in research and experimentation.