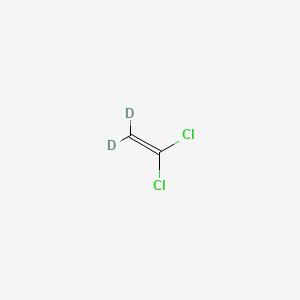
1,1-DICHLOROETHYLENE-D2
Overview
Description
1,1-Dichloroethylene-d2 (C₂D₂Cl₂) is a deuterated analog of 1,1-dichloroethylene, where two hydrogen atoms are replaced by deuterium isotopes. This substitution significantly impacts its physical and chemical properties, making it valuable in spectroscopic studies, environmental tracing, and reaction mechanism elucidation . Key characteristics include:
- Molecular weight: 98.956 g/mol (vs. 96.938 g/mol for the non-deuterated form) .
- Isotopic purity: ≥98 atom% deuterium, as standardized by suppliers like Kanto Reagents .
- CAS Registry Number: 49125-25 (for the deuterated form) .
Its structure (CD₂=CCl₂) retains the planar geometry and electron-withdrawing chlorine substituents of the parent compound, but the deuterium substitution alters vibrational frequencies and thermodynamic stability, critical in nuclear magnetic resonance (NMR) and mass spectrometry applications .
Preparation Methods
1,1-Dichloroethylene-D2 is typically synthesized through the dehydrochlorination of 1,1,2-trichloroethane-D2. This reaction is catalyzed by bases such as sodium hydroxide or calcium hydroxide at elevated temperatures (around 100°C) . The reaction can be represented as follows:
Cl2CHCH2Cl+NaOH→Cl2C=CH2+NaCl+H2O
Chemical Reactions Analysis
1,1-Dichloroethylene-D2 undergoes various chemical reactions, including:
Oxidation: It can be oxidized to form chlorinated acetic acids.
Reduction: It can be reduced to form ethylene derivatives.
Substitution: It can undergo substitution reactions with nucleophiles to form various substituted products.
Common reagents used in these reactions include oxidizing agents like potassium permanganate, reducing agents like lithium aluminum hydride, and nucleophiles like sodium methoxide. The major products formed depend on the specific reaction conditions and reagents used.
Scientific Research Applications
1,1-Dichloroethylene-D2 is widely used in scientific research due to its isotopic labeling. Some of its applications include:
Chemistry: Used as a tracer in reaction mechanisms and kinetic studies.
Biology: Employed in metabolic studies to trace the incorporation of deuterium into biological molecules.
Medicine: Utilized in pharmacokinetic studies to understand the metabolism and distribution of drugs.
Industry: Used in the production of high-purity silicon dioxide films in semiconductor device fabrication
Mechanism of Action
The mechanism of action of 1,1-Dichloroethylene-D2 involves its interaction with various molecular targets. It is metabolized in the liver to form reactive intermediates that can bind to cellular macromolecules, leading to hepatotoxicity. The primary pathway involves the formation of monochloroacetic acid, which inhibits mitochondrial function and leads to the accumulation of citric acid .
Comparison with Similar Compounds
Structural and Isotopic Differences
Table 1: Key Properties of Chlorinated Ethylenes
Compound | Molecular Formula | Molecular Weight (g/mol) | CAS RN | Isotopic Composition | Boiling Point (°C) |
---|---|---|---|---|---|
1,1-Dichloroethylene-d2 | C₂D₂Cl₂ | 98.956 | 49125-25 | 98 atom% D | ~31–33 (estimated) |
1,1-Dichloroethylene | C₂H₂Cl₂ | 96.938 | 75-35-4 | Natural abundance | 31.7 |
1,2-Dichloroethylene-d2 | C₂D₂Cl₂ | 98.956 | 49125-26 | 98 atom% D | ~48–50 (estimated) |
cis-1,2-Dichloroethylene | C₂H₂Cl₂ | 96.938 | 156-59-2 | Natural abundance | 60.1 |
trans-1,2-Dichloroethylene | C₂H₂Cl₂ | 96.938 | 156-60-5 | Natural abundance | 47.5 |
Notes:
- Deuterated forms exhibit higher molecular weights and altered boiling points due to isotopic mass effects .
- The cis and trans isomers of 1,2-dichloroethylene differ in polarity and reactivity; deuterated analogs retain these trends .
Chemical Reactivity and Stability
- Electrophilic Addition: this compound undergoes slower addition reactions compared to the non-deuterated form due to kinetic isotope effects (KIEs) .
- Thermal Decomposition: Deuterated compounds generally exhibit higher thermal stability. For example, this compound decomposes at ~200°C, whereas the non-deuterated form decomposes at ~180°C .
- Photochemical Reactivity : The C–D bond’s lower zero-point energy reduces UV-induced bond cleavage rates compared to C–H bonds .
Analytical Chemistry
- NMR Spectroscopy : The deuterium in this compound simplifies proton decoupling in ¹³C NMR, enhancing signal resolution for adjacent carbons .
- Environmental Tracing : Used as an internal standard in gas chromatography-mass spectrometry (GC-MS) to quantify volatile chlorinated hydrocarbons in water and air samples .
Toxicological Profiles
While 1,1-dichloroethylene is classified as a hazardous air pollutant (EPA) and carcinogen, its deuterated form is primarily used in controlled laboratory settings, minimizing exposure risks . In contrast, 1,1-dichloroethane (CAS 75-34-3), a structurally similar compound with single C–C bonds, shows distinct toxicokinetics, including higher water solubility (1.2 g/L) and lower volatility .
Biological Activity
1,1-Dichloroethylene-D2 (DCE-D2) is a deuterated form of 1,1-dichloroethylene (DCE), a volatile organic compound commonly used in industrial applications. This article explores the biological activity of DCE-D2, focusing on its metabolic pathways, toxicological effects, and implications for human health and the environment.
DCE-D2 undergoes rapid absorption and metabolism in biological systems. The primary metabolic pathway involves cytochrome P450 2E1 (CYP2E1), which converts DCE-D2 into several reactive metabolites, including dichloroethylene epoxide, 2-chloroacetyl chloride, and 2,2-dichloroacetaldehyde. These metabolites can interact with cellular macromolecules, leading to covalent binding and potential toxicity .
Table 1: Metabolites of this compound
Metabolite | Chemical Structure | Biological Activity |
---|---|---|
Dichloroethylene epoxide | DCE Epoxide | Highly reactive; associated with tissue damage |
2-Chloroacetyl chloride | Chloroacetyl Chloride | Potentially toxic; reacts with glutathione |
2,2-Dichloroacetaldehyde | Dichloroacetaldehyde | Involved in oxidative stress responses |
Hepatic Toxicity
Research indicates that DCE-D2 exposure can lead to significant hepatic toxicity. In rodent studies, administration of DCE-D2 resulted in elevated levels of liver enzymes such as aspartate aminotransferase (AST) and alanine aminotransferase (ALT), indicating hepatocellular damage. For instance, a study demonstrated that a single oral dose of DCE-D2 at 400 mg/kg caused a dramatic increase in serum enzyme levels within hours post-exposure .
Renal Toxicity
The kidneys also exhibit sensitivity to DCE-D2. Histopathological examinations have shown renal nephrosis and tubular necrosis in exposed animals. A notable study reported that male mice exposed to concentrations as low as 10 ppm experienced significant renal damage .
Table 2: Summary of Toxicological Findings
Study Reference | Dose (mg/kg or ppm) | Observed Effects |
---|---|---|
Jenkins and Andersen (1978) | 400 mg/kg | Increased liver enzymes |
Reynolds et al. (1984) | 200 mg/kg | Hepatic dilatation and necrosis |
Reitz et al. (1980) | 200 ppm | Severe liver morphological changes |
Case Studies and Environmental Impact
DCE-D2 is also relevant in environmental contexts due to its presence in groundwater contamination scenarios. Case studies indicate that DCE can migrate through soil and impact water supplies, posing risks to both human health and ecological systems. The U.S. Environmental Protection Agency (EPA) has documented several instances where groundwater remediation efforts were necessary due to DCE contamination .
Q & A
Basic Research Questions
Q. What are the recommended methods for synthesizing 1,1-Dichloroethylene-D₂ with high isotopic purity?
To achieve high isotopic purity (≥98 atom% D), deuteration is typically performed via catalytic exchange reactions or halogen-deuterium substitution under controlled conditions. For example, chlorinated precursors like 1,1-dichloroethylene can react with deuterium gas (D₂) in the presence of palladium or platinum catalysts at elevated temperatures (100–150°C) . Post-synthesis purification via fractional distillation or preparative gas chromatography is critical to isolate the deuterated compound from residual solvents or non-deuterated byproducts. Analytical validation using NMR (¹H and ²H) and mass spectrometry ensures isotopic integrity .
Q. How do researchers characterize the physicochemical properties of 1,1-Dichloroethylene-D₂?
Key properties include vapor pressure, boiling point, and solubility, which are determined using techniques like gas chromatography-mass spectrometry (GC-MS) and headspace analysis. For example, GC-MS with electron ionization (EI) can differentiate isotopic variants by mass-to-charge ratios (e.g., m/z 98 for non-deuterated vs. m/z 100 for deuterated species) . Thermodynamic stability under varying pH and temperature is assessed via accelerated degradation studies, monitored using Fourier-transform infrared spectroscopy (FTIR) to track C-D bond integrity .
Q. What analytical techniques are most effective for distinguishing 1,1-Dichloroethylene-D₂ from its structural isomers?
High-resolution nuclear magnetic resonance (NMR) spectroscopy is essential. The deuterium isotope effect causes distinct chemical shifts in ¹³C NMR spectra, particularly for carbons adjacent to deuterated positions. For instance, the C-Cl₂ group in 1,1-Dichloroethylene-D₂ shows a downfield shift compared to non-deuterated analogs . Gas chromatography coupled with isotope-ratio mass spectrometry (GC-IRMS) further resolves isotopic variants from isomers like 1,2-dichloroethylene-d₂ .
Advanced Research Questions
Q. How can researchers resolve contradictions in spectral data for 1,1-Dichloroethylene-D₂ across different experimental conditions?
Discrepancies in NMR or IR spectra often arise from solvent effects or isotopic scrambling. For example, polar solvents like DMSO-d₆ may induce partial proton exchange, altering observed ²H NMR signals. To mitigate this, use non-polar solvents (e.g., CDCl₃) and validate results against computational models (e.g., density functional theory (DFT) simulations of vibrational modes) . Cross-referencing with X-ray crystallography data (if crystalline derivatives are available) provides additional structural confirmation .
Q. What strategies optimize the yield of 1,1-Dichloroethylene-D₂ in multi-step syntheses involving halogenated intermediates?
Yield optimization requires minimizing side reactions such as β-elimination or over-deuteration. Reaction parameters like temperature, catalyst loading, and deuterium gas pressure must be systematically calibrated. For instance, lower temperatures (80–100°C) reduce decomposition of halogenated intermediates, while excess D₂ ensures complete deuteration without side-chain modifications . Kinetic studies using in-situ monitoring (e.g., Raman spectroscopy) identify rate-limiting steps for process refinement .
Q. How do environmental factors influence the degradation pathways of 1,1-Dichloroethylene-D₂ in aqueous systems?
Degradation studies in simulated environmental matrices (e.g., pH-buffered aqueous solutions) reveal hydrolysis and photolytic pathways. Under UV light, C-D bonds exhibit slower cleavage rates compared to C-H bonds, as shown by isotopic labeling experiments using LC-HRMS . Anaerobic microbial degradation studies (e.g., with Dehalococcoides spp.) can further elucidate reductive dechlorination mechanisms specific to deuterated compounds .
Q. What are the challenges in detecting trace impurities in 1,1-Dichloroethylene-D₂, and how are they addressed?
Trace impurities (e.g., residual chlorinated solvents or deuterium-depleted byproducts) require ultrasensitive detection methods. Comprehensive two-dimensional gas chromatography (GC×GC) paired with time-of-flight mass spectrometry (TOF-MS) enhances peak resolution for low-abundance species . Isotope dilution assays using deuterated internal standards improve quantification accuracy, particularly for regulatory compliance in environmental or toxicological studies .
Properties
IUPAC Name |
1,1-dichloro-2,2-dideuterioethene | |
---|---|---|
Source | PubChem | |
URL | https://pubchem.ncbi.nlm.nih.gov | |
Description | Data deposited in or computed by PubChem | |
InChI |
InChI=1S/C2H2Cl2/c1-2(3)4/h1H2/i1D2 | |
Source | PubChem | |
URL | https://pubchem.ncbi.nlm.nih.gov | |
Description | Data deposited in or computed by PubChem | |
InChI Key |
LGXVIGDEPROXKC-DICFDUPASA-N | |
Source | PubChem | |
URL | https://pubchem.ncbi.nlm.nih.gov | |
Description | Data deposited in or computed by PubChem | |
Canonical SMILES |
C=C(Cl)Cl | |
Source | PubChem | |
URL | https://pubchem.ncbi.nlm.nih.gov | |
Description | Data deposited in or computed by PubChem | |
Isomeric SMILES |
[2H]C(=C(Cl)Cl)[2H] | |
Source | PubChem | |
URL | https://pubchem.ncbi.nlm.nih.gov | |
Description | Data deposited in or computed by PubChem | |
Molecular Formula |
C2H2Cl2 | |
Source | PubChem | |
URL | https://pubchem.ncbi.nlm.nih.gov | |
Description | Data deposited in or computed by PubChem | |
Molecular Weight |
98.95 g/mol | |
Source | PubChem | |
URL | https://pubchem.ncbi.nlm.nih.gov | |
Description | Data deposited in or computed by PubChem | |
CAS No. |
22280-73-5 | |
Record name | Ethene-1,1-d2, 2,2-dichloro- | |
Source | CAS Common Chemistry | |
URL | https://commonchemistry.cas.org/detail?cas_rn=22280-73-5 | |
Description | CAS Common Chemistry is an open community resource for accessing chemical information. Nearly 500,000 chemical substances from CAS REGISTRY cover areas of community interest, including common and frequently regulated chemicals, and those relevant to high school and undergraduate chemistry classes. This chemical information, curated by our expert scientists, is provided in alignment with our mission as a division of the American Chemical Society. | |
Explanation | The data from CAS Common Chemistry is provided under a CC-BY-NC 4.0 license, unless otherwise stated. | |
Record name | Ethene-1,1-d2, 2,2-dichloro- | |
Source | ChemIDplus | |
URL | https://pubchem.ncbi.nlm.nih.gov/substance/?source=chemidplus&sourceid=0022280735 | |
Description | ChemIDplus is a free, web search system that provides access to the structure and nomenclature authority files used for the identification of chemical substances cited in National Library of Medicine (NLM) databases, including the TOXNET system. | |
Synthesis routes and methods I
Procedure details
Synthesis routes and methods II
Procedure details
Synthesis routes and methods III
Procedure details
Retrosynthesis Analysis
AI-Powered Synthesis Planning: Our tool employs the Template_relevance Pistachio, Template_relevance Bkms_metabolic, Template_relevance Pistachio_ringbreaker, Template_relevance Reaxys, Template_relevance Reaxys_biocatalysis model, leveraging a vast database of chemical reactions to predict feasible synthetic routes.
One-Step Synthesis Focus: Specifically designed for one-step synthesis, it provides concise and direct routes for your target compounds, streamlining the synthesis process.
Accurate Predictions: Utilizing the extensive PISTACHIO, BKMS_METABOLIC, PISTACHIO_RINGBREAKER, REAXYS, REAXYS_BIOCATALYSIS database, our tool offers high-accuracy predictions, reflecting the latest in chemical research and data.
Strategy Settings
Precursor scoring | Relevance Heuristic |
---|---|
Min. plausibility | 0.01 |
Model | Template_relevance |
Template Set | Pistachio/Bkms_metabolic/Pistachio_ringbreaker/Reaxys/Reaxys_biocatalysis |
Top-N result to add to graph | 6 |
Feasible Synthetic Routes
Disclaimer and Information on In-Vitro Research Products
Please be aware that all articles and product information presented on BenchChem are intended solely for informational purposes. The products available for purchase on BenchChem are specifically designed for in-vitro studies, which are conducted outside of living organisms. In-vitro studies, derived from the Latin term "in glass," involve experiments performed in controlled laboratory settings using cells or tissues. It is important to note that these products are not categorized as medicines or drugs, and they have not received approval from the FDA for the prevention, treatment, or cure of any medical condition, ailment, or disease. We must emphasize that any form of bodily introduction of these products into humans or animals is strictly prohibited by law. It is essential to adhere to these guidelines to ensure compliance with legal and ethical standards in research and experimentation.