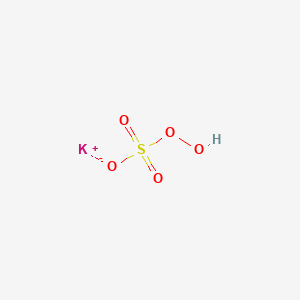
Monopotassium peroxymonosulfate
- Click on QUICK INQUIRY to receive a quote from our team of experts.
- With the quality product at a COMPETITIVE price, you can focus more on your research.
Overview
Description
It is the potassium salt of peroxymonosulfuric acid and is often available as a triple salt mixture with potassium hydrogen sulfate and potassium sulfate, known commercially as Oxone . This compound is known for its high oxidative potential and stability, making it a valuable reagent in both industrial and laboratory settings.
Mechanism of Action
Target of Action
Monopotassium peroxymonosulfate, also known as Potassium hydrogenperoxomonosulphate, is a versatile oxidizing agent . Its primary targets are various organic and inorganic substances that can be oxidized, including aldehydes, internal alkenes, sulfides, tertiary amines, and phosphines .
Mode of Action
This compound interacts with its targets through oxidation . It can oxidize aldehydes to carboxylic acids, internal alkenes may be cleaved to two carboxylic acids, sulfides give sulfones, tertiary amines give amine oxides, and phosphines give phosphine oxides . The standard electrode potential for this compound is +1.81 V with a half reaction generating the hydrogen sulfate .
Biochemical Pathways
This compound affects various biochemical pathways through its oxidation process . The reactive oxygen species (ROS) produced by activating this compound include powerful oxidants such as sulfate radical (SO·−4), hydroxyl radical (OH), superoxide radical (O·−2), and singlet oxygen (1 O 2) . These ROS can effectively degrade and mineralize various organic pollutants .
Pharmacokinetics
Its solubility in water and its decomposition in aqueous solutions are key factors influencing its bioavailability .
Result of Action
The result of this compound’s action is the oxidation of target substances, leading to their transformation or degradation . For example, it can convert aldehydes to carboxylic acids or esters, cleave internal alkenes to two carboxylic acids, and transform sulfides into sulfones .
Action Environment
The action of this compound is influenced by various environmental factors. Its stability reaches a minimum at pH 9 . Transition metals like iron, cobalt, nickel, copper, manganese can catalyze the decay of this compound in solution . Moreover, it is sensitive to heat, with decomposition to SO 2 and SO 3 starting at 300°C . In terms of storage, as long as this compound is stored under dry and cool conditions, it loses about 1% activity per month under release of oxygen and heat .
Biochemical Analysis
Biochemical Properties
Monopotassium peroxymonosulfate plays a significant role in biochemical reactions due to its strong oxidizing properties . It interacts with various biomolecules, including enzymes and proteins, through oxidation reactions . These interactions often result in the modification of these biomolecules, altering their function and activity .
Cellular Effects
The effects of this compound on cells and cellular processes are primarily due to its oxidative properties . It can influence cell function by modifying cellular components through oxidation, potentially impacting cell signaling pathways, gene expression, and cellular metabolism .
Molecular Mechanism
This compound exerts its effects at the molecular level through its strong oxidizing properties . It can interact with biomolecules through binding interactions, leading to the oxidation of these molecules . This can result in enzyme inhibition or activation, and changes in gene expression .
Temporal Effects in Laboratory Settings
In laboratory settings, the effects of this compound can change over time . It is known to have a long shelf life and loses less than 1% of its oxidizing power per month . Information on its stability, degradation, and long-term effects on cellular function in in vitro or in vivo studies is currently limited .
Metabolic Pathways
This compound is involved in various oxidative reactions in metabolic pathways
Preparation Methods
Synthetic Routes and Reaction Conditions: Monopotassium peroxymonosulfate is synthesized from peroxysulfuric acid, which is generated in situ by combining oleum (fuming sulfuric acid) and hydrogen peroxide. The resulting solution is carefully neutralized with potassium hydroxide, allowing the crystallization of the triple salt mixture .
Industrial Production Methods: In industrial settings, the production of this compound follows a similar process. The key steps involve the controlled reaction of oleum and hydrogen peroxide to form peroxysulfuric acid, followed by neutralization with potassium hydroxide. The crystallized product is then filtered, washed, and dried to obtain the final compound .
Chemical Reactions Analysis
Types of Reactions: Monopotassium peroxymonosulfate primarily undergoes oxidation reactions due to its strong oxidative properties. It can oxidize a wide range of organic and inorganic compounds.
Common Reagents and Conditions:
Oxidation of Aldehydes to Carboxylic Acids: this compound can oxidize aldehydes to carboxylic acids in the presence of water or alcoholic solvents.
Epoxidation of Alkenes: It can epoxidize terminal alkenes, converting them into epoxides.
Oxidation of Sulfides to Sulfones: Sulfides can be oxidized to sulfones using this compound.
Oxidation of Tertiary Amines to Amine Oxides: Tertiary amines can be converted to amine oxides.
Major Products Formed:
- Carboxylic acids from aldehydes
- Epoxides from alkenes
- Sulfones from sulfides
- Amine oxides from tertiary amines
Scientific Research Applications
Monopotassium peroxymonosulfate has a wide range of applications in scientific research:
Comparison with Similar Compounds
Potassium Persulfate: Another strong oxidizing agent used in similar applications but with different reactivity and stability profiles.
Sodium Percarbonate: Commonly used as a bleaching agent and in cleaning products, it releases hydrogen peroxide upon dissolution in water.
Uniqueness: Monopotassium peroxymonosulfate is unique due to its high oxidative potential, stability, and versatility in various applications. Its ability to generate multiple types of reactive oxygen species makes it particularly effective in oxidation reactions and disinfection processes .
Properties
CAS No. |
10058-23-8 |
---|---|
Molecular Formula |
HKO5S |
Molecular Weight |
152.17 g/mol |
IUPAC Name |
potassium;oxido hydrogen sulfate |
InChI |
InChI=1S/K.H2O5S/c;1-5-6(2,3)4/h;1H,(H,2,3,4)/q+1;/p-1 |
InChI Key |
OKBMCNHOEMXPTM-UHFFFAOYSA-M |
SMILES |
OOS(=O)(=O)[O-].[K+] |
Canonical SMILES |
OS(=O)(=O)O[O-].[K+] |
Key on ui other cas no. |
10058-23-8 |
Origin of Product |
United States |
Disclaimer and Information on In-Vitro Research Products
Please be aware that all articles and product information presented on BenchChem are intended solely for informational purposes. The products available for purchase on BenchChem are specifically designed for in-vitro studies, which are conducted outside of living organisms. In-vitro studies, derived from the Latin term "in glass," involve experiments performed in controlled laboratory settings using cells or tissues. It is important to note that these products are not categorized as medicines or drugs, and they have not received approval from the FDA for the prevention, treatment, or cure of any medical condition, ailment, or disease. We must emphasize that any form of bodily introduction of these products into humans or animals is strictly prohibited by law. It is essential to adhere to these guidelines to ensure compliance with legal and ethical standards in research and experimentation.