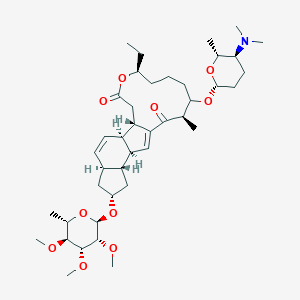
Spinosyn A
Overview
Description
Spinosyn A is a naturally occurring compound produced by the bacterium Saccharopolyspora spinosa. It is a member of the spinosyn family, which are macrolide antibiotics with potent insecticidal properties. This compound, along with its isomer Spinosyn D, is a key component of the insecticide Spinosad. These compounds are known for their unique chemical structure and their effectiveness in controlling a wide range of insect pests while being relatively non-toxic to mammals and other non-target organisms .
Mechanism of Action
Target of Action
Spinosyn A, a component of the insecticide Spinosad, primarily targets the nicotinic acetylcholine receptors (nAChRs) of the insect nervous system . These receptors play a crucial role in the transmission of nerve impulses. The interaction of this compound with these receptors is unique and distinct from the interaction sites of other insecticides .
Mode of Action
Instead, it acts via a novel mechanism that resembles a GABA antagonist . This interaction leads to the disruption of acetylcholine neurotransmission , causing involuntary muscle contractions and paralysis .
Biochemical Pathways
The biosynthetic pathway of this compound has been elucidated through precursor-labeling studies, analysis of biosynthetically blocked mutants, and in vitro analysis of enzymes involved in this compound biosynthesis . The compound is produced by the bacterial species Saccharopolyspora spinosa, which was discovered in isolates from crushed sugarcane .
Result of Action
The primary result of this compound’s action is potent insecticidal activity. It is effective against many commercially significant species that cause extensive damage to crops and other plants . Additionally, it shows activity against important external parasites of livestock, companion animals, and humans .
Action Environment
This compound is derived from fermentation, a green process that uses renewable agricultural feedstocks . The compound has a short environmental persistence and degrades into simpler fragments containing only carbon, oxygen, nitrogen, and hydrogen . Its insecticidal spectrum, unique mode of action, and lower environmental effect make it a useful agent for modern integrated pest management programs .
Biochemical Analysis
Biochemical Properties
Spinosyn A interacts with various biomolecules in its biochemical reactions. It is known to disrupt nicotinic acetylcholine receptors . In the context of cancer biology, this compound has been found to bind to and activate Argininosuccinate Synthase (ASS1), an enzyme that catalyzes the formation of argininosuccinate from citrulline and aspartate . This interaction is critical for the anti-cancer effects of this compound .
Cellular Effects
This compound has significant effects on various types of cells and cellular processes. In breast cancer cells, it has been shown to inhibit cell proliferation and migration, induce apoptosis, and cause cell cycle arrest . It also modulates multiple signaling pathways, including the PI3K/Akt/mTOR pathway, the MAPK/ERK pathway, and the JAK/STAT pathway .
Molecular Mechanism
The molecular mechanism of this compound involves its binding interactions with biomolecules and changes in gene expression. It functions as an allosteric activator of nicotinic acetylcholine receptors . In cancer cells, this compound binds to and activates ASS1, leading to reduced pyrimidine synthesis and consequently the inhibition of cancer cell proliferation .
Temporal Effects in Laboratory Settings
The effects of this compound change over time in laboratory settings. While specific studies detailing the temporal effects of this compound are limited, it is known that the compound has long-term effects on cellular function, such as inhibiting cell proliferation and inducing apoptosis in cancer cells .
Metabolic Pathways
This compound is involved in various metabolic pathways. It is known to impact the urea cycle through its interaction with ASS1 . Detailed information on the specific metabolic pathways that this compound is involved in is currently limited.
Preparation Methods
Synthetic Routes and Reaction Conditions: Spinosyn A is primarily produced through the fermentation of Saccharopolyspora spinosa. The fermentation process involves culturing the bacterium in a nutrient-rich medium under controlled conditions. The production yield can be enhanced through genetic manipulation and optimization of fermentation parameters. For example, genome shuffling and antibiotic resistance screening have been used to develop high-yield strains of Saccharopolyspora spinosa .
Industrial Production Methods: Industrial production of this compound involves large-scale fermentation followed by extraction and purification. The fermentation broth is typically subjected to solvent extraction to isolate the spinosyn compounds. The crude extract is then purified using chromatographic techniques to obtain pure this compound. The process is designed to maximize yield and ensure the purity of the final product .
Chemical Reactions Analysis
Types of Reactions: Spinosyn A undergoes various chemical reactions, including oxidation, reduction, and substitution. One notable reaction is the Diels-Alder reaction, which is catalyzed by an enzyme in Saccharopolyspora spinosa. This reaction is a key step in the biosynthesis of this compound .
Common Reagents and Conditions: Common reagents used in the chemical modification of this compound include oxidizing agents, reducing agents, and nucleophiles. Reaction conditions typically involve controlled temperatures and pH levels to ensure the desired chemical transformations. For example, oxidation reactions may be carried out using reagents such as potassium permanganate or hydrogen peroxide under acidic or basic conditions .
Major Products Formed: The major products formed from the chemical reactions of this compound include various derivatives with modified functional groups. These derivatives can exhibit different biological activities and are often studied for their potential use as insecticides or pharmaceuticals .
Scientific Research Applications
Spinosyn A has a wide range of scientific research applications. In chemistry, it is studied for its unique chemical structure and its potential as a lead compound for the development of new insecticides. In biology, this compound is used to study the mechanisms of insect resistance and the interactions between insecticides and their targets. In medicine, this compound and its derivatives are investigated for their potential use as antibiotics and antiparasitic agents. In industry, this compound is used in the formulation of insecticides for agricultural and veterinary applications .
Comparison with Similar Compounds
Similar Compounds: Similar compounds to Spinosyn A include Spinosyn D, Spinosyn E, and Spinosyn F. These compounds share a similar macrolide structure but differ in the degree of methylation and other functional group modifications .
Uniqueness of this compound: this compound is unique due to its high insecticidal potency and its relatively low toxicity to non-target organisms. Its unique mechanism of action and its effectiveness against resistant insect populations make it a valuable tool in integrated pest management programs. Additionally, the ability to produce this compound through fermentation provides a sustainable and scalable method for its production .
Properties
IUPAC Name |
(1S,2R,5S,7R,9R,10S,14R,15S,19S)-15-[(2R,5S,6R)-5-(dimethylamino)-6-methyloxan-2-yl]oxy-19-ethyl-14-methyl-7-[(2R,3R,4R,5S,6S)-3,4,5-trimethoxy-6-methyloxan-2-yl]oxy-20-oxatetracyclo[10.10.0.02,10.05,9]docosa-3,11-diene-13,21-dione | |
---|---|---|
Source | PubChem | |
URL | https://pubchem.ncbi.nlm.nih.gov | |
Description | Data deposited in or computed by PubChem | |
InChI |
InChI=1S/C41H65NO10/c1-10-26-12-11-13-34(52-36-17-16-33(42(5)6)23(3)48-36)22(2)37(44)32-20-30-28(31(32)21-35(43)50-26)15-14-25-18-27(19-29(25)30)51-41-40(47-9)39(46-8)38(45-7)24(4)49-41/h14-15,20,22-31,33-34,36,38-41H,10-13,16-19,21H2,1-9H3/t22-,23-,24+,25-,26+,27-,28-,29-,30-,31+,33+,34+,36+,38+,39-,40-,41+/m1/s1 | |
Source | PubChem | |
URL | https://pubchem.ncbi.nlm.nih.gov | |
Description | Data deposited in or computed by PubChem | |
InChI Key |
SRJQTHAZUNRMPR-UYQKXTDMSA-N | |
Source | PubChem | |
URL | https://pubchem.ncbi.nlm.nih.gov | |
Description | Data deposited in or computed by PubChem | |
Canonical SMILES |
CCC1CCCC(C(C(=O)C2=CC3C4CC(CC4C=CC3C2CC(=O)O1)OC5C(C(C(C(O5)C)OC)OC)OC)C)OC6CCC(C(O6)C)N(C)C | |
Source | PubChem | |
URL | https://pubchem.ncbi.nlm.nih.gov | |
Description | Data deposited in or computed by PubChem | |
Isomeric SMILES |
CC[C@H]1CCC[C@@H]([C@H](C(=O)C2=C[C@H]3[C@@H]4C[C@@H](C[C@H]4C=C[C@H]3[C@@H]2CC(=O)O1)O[C@H]5[C@@H]([C@@H]([C@H]([C@@H](O5)C)OC)OC)OC)C)O[C@H]6CC[C@@H]([C@H](O6)C)N(C)C | |
Source | PubChem | |
URL | https://pubchem.ncbi.nlm.nih.gov | |
Description | Data deposited in or computed by PubChem | |
Molecular Formula |
C41H65NO10 | |
Source | PubChem | |
URL | https://pubchem.ncbi.nlm.nih.gov | |
Description | Data deposited in or computed by PubChem | |
DSSTOX Substance ID |
DTXSID8037598 | |
Record name | Spinosyn A | |
Source | EPA DSSTox | |
URL | https://comptox.epa.gov/dashboard/DTXSID8037598 | |
Description | DSSTox provides a high quality public chemistry resource for supporting improved predictive toxicology. | |
Molecular Weight |
732.0 g/mol | |
Source | PubChem | |
URL | https://pubchem.ncbi.nlm.nih.gov | |
Description | Data deposited in or computed by PubChem | |
Physical Description |
White odorless solid; [Merck Index] | |
Record name | Spinosyn A | |
Source | Haz-Map, Information on Hazardous Chemicals and Occupational Diseases | |
URL | https://haz-map.com/Agents/7193 | |
Description | Haz-Map® is an occupational health database designed for health and safety professionals and for consumers seeking information about the adverse effects of workplace exposures to chemical and biological agents. | |
Explanation | Copyright (c) 2022 Haz-Map(R). All rights reserved. Unless otherwise indicated, all materials from Haz-Map are copyrighted by Haz-Map(R). No part of these materials, either text or image may be used for any purpose other than for personal use. Therefore, reproduction, modification, storage in a retrieval system or retransmission, in any form or by any means, electronic, mechanical or otherwise, for reasons other than personal use, is strictly prohibited without prior written permission. | |
Solubility |
Water = 89.4 ppm (Spinosyn A) and 0.495 ppm (Spinosyn D); acetone - 16.8 g/ml (Spinosyn A) and 1.01 g/ml (Spinosyn D); methanol - 19.0 g/ml (Spinosyn A) and 0.252 g/ml (Spinosyn D) | |
Record name | SPINOSYN-A | |
Source | Hazardous Substances Data Bank (HSDB) | |
URL | https://pubchem.ncbi.nlm.nih.gov/source/hsdb/7006 | |
Description | The Hazardous Substances Data Bank (HSDB) is a toxicology database that focuses on the toxicology of potentially hazardous chemicals. It provides information on human exposure, industrial hygiene, emergency handling procedures, environmental fate, regulatory requirements, nanomaterials, and related areas. The information in HSDB has been assessed by a Scientific Review Panel. | |
Density |
The electrophilic addition of reagents to the 5,6-double bond in spinosyn A & spinosyn D systems occurred with high pi-diastereofacial selectivity. Addition occurred preferentially from the beta face of the molecule with selectivities ranging from 5:1 to better than 30:1. Various NMR properties were investigated in order to distinguish the beta & alpha isomers with the help of theoretical models of the products. These NMR properties include a (13)C gamma effect to C-11 & vicinal coupling between H-4 & H-5. To help rationalize the selectivity, computational studies on the transition states for epoxidation were calculated using density functional theory. The results indicate that beta epoxidation is favored & that the geometries of the transition structures are consistent with torsional steering being the source of the selectivity. | |
Record name | SPINOSYN-A | |
Source | Hazardous Substances Data Bank (HSDB) | |
URL | https://pubchem.ncbi.nlm.nih.gov/source/hsdb/7006 | |
Description | The Hazardous Substances Data Bank (HSDB) is a toxicology database that focuses on the toxicology of potentially hazardous chemicals. It provides information on human exposure, industrial hygiene, emergency handling procedures, environmental fate, regulatory requirements, nanomaterials, and related areas. The information in HSDB has been assessed by a Scientific Review Panel. | |
Vapor Pressure |
3.0X10-11 kPA at 25 C (Spinosyn A) | |
Record name | SPINOSYN-A | |
Source | Hazardous Substances Data Bank (HSDB) | |
URL | https://pubchem.ncbi.nlm.nih.gov/source/hsdb/7006 | |
Description | The Hazardous Substances Data Bank (HSDB) is a toxicology database that focuses on the toxicology of potentially hazardous chemicals. It provides information on human exposure, industrial hygiene, emergency handling procedures, environmental fate, regulatory requirements, nanomaterials, and related areas. The information in HSDB has been assessed by a Scientific Review Panel. | |
Mechanism of Action |
The spinosyns, a novel family of insecticidal macrocyclic lactones, are active on a wide variety of insect pests, especially lepidopterans & dipterans...& possess a mode of action that appears unique, with studies to date suggesting that both nicotinic & gamma-aminobutryic acid receptor functions are altered in a novel manner. Compared to pyrethroids such as cypermethrin, spinosyn A is slow to penetrate into insect larvae such as tobacco budworm larvae (Heliothis virescens); however, once inside the insect, spinosyn A is not readily metabolized... | |
Record name | SPINOSYN-A | |
Source | Hazardous Substances Data Bank (HSDB) | |
URL | https://pubchem.ncbi.nlm.nih.gov/source/hsdb/7006 | |
Description | The Hazardous Substances Data Bank (HSDB) is a toxicology database that focuses on the toxicology of potentially hazardous chemicals. It provides information on human exposure, industrial hygiene, emergency handling procedures, environmental fate, regulatory requirements, nanomaterials, and related areas. The information in HSDB has been assessed by a Scientific Review Panel. | |
Color/Form |
Light grey to white crystals | |
CAS No. |
131929-60-7 | |
Record name | Spinosyn A | |
Source | CAS Common Chemistry | |
URL | https://commonchemistry.cas.org/detail?cas_rn=131929-60-7 | |
Description | CAS Common Chemistry is an open community resource for accessing chemical information. Nearly 500,000 chemical substances from CAS REGISTRY cover areas of community interest, including common and frequently regulated chemicals, and those relevant to high school and undergraduate chemistry classes. This chemical information, curated by our expert scientists, is provided in alignment with our mission as a division of the American Chemical Society. | |
Explanation | The data from CAS Common Chemistry is provided under a CC-BY-NC 4.0 license, unless otherwise stated. | |
Record name | Spinosad factor A [USAN] | |
Source | ChemIDplus | |
URL | https://pubchem.ncbi.nlm.nih.gov/substance/?source=chemidplus&sourceid=0131929607 | |
Description | ChemIDplus is a free, web search system that provides access to the structure and nomenclature authority files used for the identification of chemical substances cited in National Library of Medicine (NLM) databases, including the TOXNET system. | |
Record name | Spinosyn A | |
Source | EPA DSSTox | |
URL | https://comptox.epa.gov/dashboard/DTXSID8037598 | |
Description | DSSTox provides a high quality public chemistry resource for supporting improved predictive toxicology. | |
Record name | spinosyn A | |
Source | European Chemicals Agency (ECHA) | |
URL | https://echa.europa.eu/information-on-chemicals | |
Description | The European Chemicals Agency (ECHA) is an agency of the European Union which is the driving force among regulatory authorities in implementing the EU's groundbreaking chemicals legislation for the benefit of human health and the environment as well as for innovation and competitiveness. | |
Explanation | Use of the information, documents and data from the ECHA website is subject to the terms and conditions of this Legal Notice, and subject to other binding limitations provided for under applicable law, the information, documents and data made available on the ECHA website may be reproduced, distributed and/or used, totally or in part, for non-commercial purposes provided that ECHA is acknowledged as the source: "Source: European Chemicals Agency, http://echa.europa.eu/". Such acknowledgement must be included in each copy of the material. ECHA permits and encourages organisations and individuals to create links to the ECHA website under the following cumulative conditions: Links can only be made to webpages that provide a link to the Legal Notice page. | |
Record name | SPINOSAD FACTOR A | |
Source | FDA Global Substance Registration System (GSRS) | |
URL | https://gsrs.ncats.nih.gov/ginas/app/beta/substances/OY0L59V61N | |
Description | The FDA Global Substance Registration System (GSRS) enables the efficient and accurate exchange of information on what substances are in regulated products. Instead of relying on names, which vary across regulatory domains, countries, and regions, the GSRS knowledge base makes it possible for substances to be defined by standardized, scientific descriptions. | |
Explanation | Unless otherwise noted, the contents of the FDA website (www.fda.gov), both text and graphics, are not copyrighted. They are in the public domain and may be republished, reprinted and otherwise used freely by anyone without the need to obtain permission from FDA. Credit to the U.S. Food and Drug Administration as the source is appreciated but not required. | |
Record name | SPINOSYN-A | |
Source | Hazardous Substances Data Bank (HSDB) | |
URL | https://pubchem.ncbi.nlm.nih.gov/source/hsdb/7006 | |
Description | The Hazardous Substances Data Bank (HSDB) is a toxicology database that focuses on the toxicology of potentially hazardous chemicals. It provides information on human exposure, industrial hygiene, emergency handling procedures, environmental fate, regulatory requirements, nanomaterials, and related areas. The information in HSDB has been assessed by a Scientific Review Panel. | |
Melting Point |
84 C to 99.5 C | |
Record name | SPINOSYN-A | |
Source | Hazardous Substances Data Bank (HSDB) | |
URL | https://pubchem.ncbi.nlm.nih.gov/source/hsdb/7006 | |
Description | The Hazardous Substances Data Bank (HSDB) is a toxicology database that focuses on the toxicology of potentially hazardous chemicals. It provides information on human exposure, industrial hygiene, emergency handling procedures, environmental fate, regulatory requirements, nanomaterials, and related areas. The information in HSDB has been assessed by a Scientific Review Panel. | |
Retrosynthesis Analysis
AI-Powered Synthesis Planning: Our tool employs the Template_relevance Pistachio, Template_relevance Bkms_metabolic, Template_relevance Pistachio_ringbreaker, Template_relevance Reaxys, Template_relevance Reaxys_biocatalysis model, leveraging a vast database of chemical reactions to predict feasible synthetic routes.
One-Step Synthesis Focus: Specifically designed for one-step synthesis, it provides concise and direct routes for your target compounds, streamlining the synthesis process.
Accurate Predictions: Utilizing the extensive PISTACHIO, BKMS_METABOLIC, PISTACHIO_RINGBREAKER, REAXYS, REAXYS_BIOCATALYSIS database, our tool offers high-accuracy predictions, reflecting the latest in chemical research and data.
Strategy Settings
Precursor scoring | Relevance Heuristic |
---|---|
Min. plausibility | 0.01 |
Model | Template_relevance |
Template Set | Pistachio/Bkms_metabolic/Pistachio_ringbreaker/Reaxys/Reaxys_biocatalysis |
Top-N result to add to graph | 6 |
Feasible Synthetic Routes
Disclaimer and Information on In-Vitro Research Products
Please be aware that all articles and product information presented on BenchChem are intended solely for informational purposes. The products available for purchase on BenchChem are specifically designed for in-vitro studies, which are conducted outside of living organisms. In-vitro studies, derived from the Latin term "in glass," involve experiments performed in controlled laboratory settings using cells or tissues. It is important to note that these products are not categorized as medicines or drugs, and they have not received approval from the FDA for the prevention, treatment, or cure of any medical condition, ailment, or disease. We must emphasize that any form of bodily introduction of these products into humans or animals is strictly prohibited by law. It is essential to adhere to these guidelines to ensure compliance with legal and ethical standards in research and experimentation.