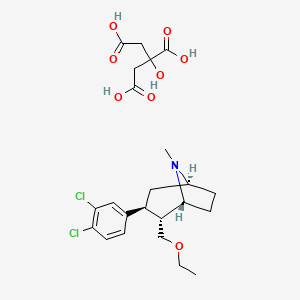
Tesofensine citrate
Overview
Description
Tesofensine citrate (C₂₃H₃₁Cl₂NO₈) is a citrate salt of tesofensine, a novel triple monoamine reuptake inhibitor (TRI) targeting dopamine (DA), norepinephrine (NE), and serotonin (5-HT) transporters . Initially developed by NeuroSearch for Alzheimer’s disease (AD) and Parkinson’s disease (PD), its clinical focus shifted to obesity after significant weight loss was observed in trials . The compound’s stereochemical complexity, with four stereocenters, contributes to its unique pharmacokinetic (PK) and pharmacodynamic (PD) properties .
Preparation Methods
Synthetic Preparation Methods of Tesofensine Citrate
Synthetic Routes and Key Chemical Steps
The synthesis of this compound involves multiple chemical steps, starting with the construction of the phenyltropane core, which is essential for its pharmacological activity.
Tropane Ring Formation: The initial step involves cyclization reactions of suitable precursors to form the bicyclic tropane ring system, a hallmark of tesofensine's structure.
Phenyl Group Introduction: The phenyl moiety is introduced via Friedel-Crafts alkylation, attaching the dichlorophenyl group to the tropane core.
Functionalization: Subsequent steps functionalize the tropane ring by adding ethoxymethyl and dichlorophenyl substituents to achieve the desired molecular configuration.
These steps are optimized in industrial settings for high yield and purity, employing efficient purification and quality control to ensure pharmaceutical-grade this compound.
Chemical Reaction Conditions and Reagents
Oxidation: Potassium permanganate (KMnO₄) under acidic conditions is used for oxidation reactions on the ethoxymethyl group.
Reduction: Lithium aluminum hydride (LiAlH₄) is employed for reduction steps, particularly for ketone intermediates.
The dichlorophenyl group, due to its electron-withdrawing nature, shows enhanced reactivity in nucleophilic aromatic substitution compared to non-halogenated analogues.
Pharmaceutical Formulation and Tablet Preparation
Composition and Excipients
The pharmaceutical preparation of this compound primarily involves formulating it into coated tablets with precise dose strengths (0.25 mg, 0.5 mg, and 1.0 mg). The key excipients used are:
Excipient | Role | Notes on Compatibility |
---|---|---|
Lactose monohydrate | Primary filler | Superior compression characteristics, compatible with active ingredient |
Microcrystalline cellulose | Filler in final blend | Enhances tablet hardness and reduces hardness variation |
Hydroxypropylcellulose | Binder | Provides resistance to crushing and granule plasticity, compatible |
Croscarmellose sodium | Disintegrant | Effective disintegrant, compatible with active ingredient |
Magnesium stearate | Lubricant | Prevents sticking during compression |
Ethanol | Co-solvent in granulation | Improves solubility and drying of granulate |
Macrogol 6000 | Plasticizer in coating | Used despite some incompatibility due to low content and polymer chain length |
Titanium dioxide | Pigment in coating | Used despite incompatibility for tablet appearance |
Iron oxide | Pigment in coating | Provides desired final tablet color |
The tablet core consists essentially of this compound and the excipients listed above, with possible residual solvents such as water or ethanol.
Preparation Process
The preparation of this compound coated tablets follows these steps:
Granulation Liquid Preparation: this compound and hydroxypropylcellulose are dissolved in a water/ethanol mixture to form the granulation liquid.
Premixing Fillers: Lactose monohydrate is premixed using an impeller.
Wet Granulation: The lactose blend is wetted by spraying the granulation liquid under continuous mixing.
Drying: The wet granulate is dried in a fluid bed dryer to remove solvents.
Sieving and Blending: The dried granulate is sieved; croscarmellose sodium and microcrystalline cellulose are also sieved and premixed with the granulate.
Final Blending: Magnesium stearate is sieved and added last to the blend to ensure lubrication.
Tableting: The blend is compressed into tablets using conventional tablet presses.
Coating: Tablets are coated with a film containing macrogol 6000 and pigments such as titanium dioxide and iron oxide.
Packaging: Final tablets are packaged in blister packs (PVC/PVCD-aluminum) or HDPE bottles for storage.
Tablet Composition Example (0.5 mg Dose)
Ingredient | Amount (mg/tablet) | Function |
---|---|---|
This compound | 0.5 | Active pharmaceutical ingredient |
Lactose monohydrate | ~260 | Filler |
Microcrystalline cellulose | ~260 | Filler |
Hydroxypropylcellulose | ~60 | Binder |
Croscarmellose sodium | ~60 | Disintegrant |
Magnesium stearate | ~5 | Lubricant |
(Note: Exact amounts vary slightly depending on formulation batch and tablet strength).
Summary Table: Preparation Methods Overview
Step | Description | Key Parameters/Notes |
---|---|---|
Synthetic Core Formation | Cyclization and functionalization of tropane ring | Use of Friedel-Crafts alkylation, oxidation/reduction steps |
Granulation | Dissolving active and binder in ethanol/water, wetting fillers | Use of ethanol co-solvent for improved solubility |
Drying | Fluid bed drying of wet granulate | Removal of solvents, control moisture content |
Blending | Mixing sieved excipients with granulate | Final addition of magnesium stearate for lubrication |
Compression | Tablet formation using conventional presses | Control of tablet hardness and uniformity |
Coating | Application of film coat with plasticizer and pigments | Use of macrogol 6000 and titanium dioxide/iron oxide |
Packaging | Blister packs or HDPE bottles | Protection from moisture and light |
Chemical Reactions Analysis
Metabolic Reactions
Tesofensine citrate undergoes hepatic metabolism primarily via cytochrome P450 3A4 (CYP3A4) . The major metabolic pathway involves N-dealkylation , producing the active metabolite M1 (desalkyl-tesofensine) .
Key Metabolic Data:
Parameter | Value | Source |
---|---|---|
Primary Enzyme | CYP3A4 | |
Major Metabolite | M1 (NS2360) | |
Half-Life (Parent) | 220 hours | |
Half-Life (M1) | 374 hours | |
Metabolite Activity | ~6% of parent compound |
M1 retains partial pharmacological activity, contributing to the compound’s sustained effects .
Degradation Under Stress Conditions
Stability studies reveal degradation pathways under accelerated storage conditions (40°C/75% RH) :
Degradation Products and Conditions:
Stability Profile in Coated Tablets :
Parameter | Initial | 6 Months (25°C) | 3 Months (40°C) |
---|---|---|---|
Dissolution Rate | 95–100% | 93–98% | 90–95% |
Degradation Products | <0.5% | <1.2% | <2.5% |
Compression Strength | 120–150 N | 110–140 N | 100–130 N |
Degradation products were quantified using HPLC, with no significant changes in appearance or assay values under standard storage .
Synthetic and Functional Group Reactivity
While synthetic routes are proprietary, structural analysis (SMILES: CCOC[C@H]1[C@H]2CC[C@@H](C[C@@H]1C3=CC(Cl)=C(Cl)C=C3)N2C
) highlights reactive sites:
Functional Group Reactivity:
Reagents and Conditions:
-
Oxidation : Potassium permanganate (KMnO₄) under acidic conditions .
-
Reduction : Lithium aluminum hydride (LiAlH₄) for ketone intermediates .
Comparative Reactivity with Analogues
Tesofensine’s dichlorophenyl group enhances electron-withdrawing effects, increasing susceptibility to nucleophilic aromatic substitution compared to non-halogenated analogues .
Pharmacological Correlations
Metabolic and degradation pathways directly impact pharmacokinetics:
Scientific Research Applications
Pharmacological Mechanism
Tesofensine citrate operates by inhibiting the reuptake of key neurotransmitters:
- Norepinephrine (NE) : Increases energy expenditure and reduces appetite.
- Serotonin (5-HT) : Enhances feelings of satiety.
- Dopamine (DA) : Influences reward pathways associated with food intake.
This mechanism positions tesofensine as a candidate for managing obesity by promoting weight loss and altering eating behaviors .
Weight Loss Efficacy
Clinical trials have demonstrated that this compound can lead to significant weight loss in obese individuals. A notable phase II trial reported:
- Participants : 203 obese patients (BMI 30-40 kg/m²).
- Duration : 24 weeks.
- Results :
These results suggest that tesofensine may produce weight loss nearly double that of existing approved weight-loss medications.
Long-term Effects
In a follow-up study (TIPO-4), patients who initially received tesofensine continued to lose weight over an extended period, indicating its potential for sustained weight management. Participants who had been on a placebo showed significant weight loss after switching to tesofensine treatment .
Safety and Tolerability
The safety profile of tesofensine has been assessed in various studies:
- Common adverse effects include dry mouth, nausea, constipation, and insomnia.
- No significant increases in blood pressure were observed at lower doses, although heart rate increased at higher doses .
Broader Therapeutic Applications
While primarily studied for obesity treatment, there is interest in the potential applications of this compound in other areas:
- Mood Disorders : Due to its action on neurotransmitter systems, there is speculation about its efficacy in treating mood disorders, although this has not been extensively researched.
- Neurological Disorders : Originally developed for Alzheimer's and Parkinson's diseases, further exploration into its neuroprotective properties could be warranted .
Comparative Analysis with Other Weight Loss Agents
Feature | This compound | Other Weight Loss Drugs |
---|---|---|
Mechanism | Triple reuptake inhibitor | Varies (e.g., appetite suppressants) |
Average Weight Loss | Up to 10.6% | Typically 3-5% |
Duration of Efficacy | Long-term potential | Short-term efficacy |
Common Side Effects | Dry mouth, nausea | Varies |
Mechanism of Action
Tesofensine citrate exerts its effects by inhibiting the reuptake of serotonin, noradrenaline, and dopamine. This leads to increased levels of these neurotransmitters in the synaptic cleft, enhancing their signaling. The primary molecular targets are the serotonin transporter, norepinephrine transporter, and dopamine transporter. This inhibition results in appetite suppression and increased energy expenditure, contributing to its anti-obesity effects .
Comparison with Similar Compounds
Mechanism of Action
Tesofensine vs. Phentermine: Tesofensine inhibits presynaptic monoamine reuptake, indirectly stimulating the cholinergic system . In contrast, phentermine primarily acts as a NE releaser, with minimal effects on DA and 5-HT . Preclinical studies show tesofensine achieves near-complete inhibition of DA reuptake (EC₅₀: 0.29 μM vs. 1.26 μM for its metabolite M1) , whereas phentermine’s mechanism lacks direct 5-HT modulation .
Tesofensine vs. Sibutramine :
Both compounds are TRIs, but sibutramine (withdrawn in 2010 due to cardiovascular risks) exhibits stronger 5-HT reuptake inhibition. Tesofensine’s metabolite M1 has fivefold lower potency than the parent compound, reducing cumulative toxicity risks .
Pharmacokinetics and Metabolism
Half-Life and Clearance :
Tesofensine and M1 have prolonged half-lives (>200 hours in humans), enabling once-daily dosing . This contrasts sharply with phentermine (19–24 hours) and sibutramine (1–2 days) . Tesofensine is metabolized via CYP3A4 to M1, which accounts for 33% of steady-state plasma concentrations .
Efficacy in Obesity and Cognitive Disorders
Weight Loss :
In phase II trials, tesofensine induced 6–12% weight loss over 6 months—double the efficacy of orlistat and sibutramine . Its dual action (appetite suppression + energy expenditure increase) contrasts with phentermine’s appetite-focused effects .
Cognitive Function : In AD trials, tesofensine improved cognitive scores (ADAS-Cog) by ~1.5 points in phase IIa, comparable to donepezil . However, a phase IIb trial (430 patients) failed to meet efficacy endpoints, likely due to disease progression overwhelming drug effects .
Biological Activity
Tesofensine citrate is a compound that has garnered attention for its potential in treating obesity and its pharmacological effects on neurotransmitters. Originally developed for neurological disorders, the focus has shifted primarily to its efficacy in weight management. This article delves into the biological activity of this compound, its mechanisms of action, pharmacokinetics, and findings from clinical studies.
This compound acts as a Serotonin-Norepinephrine-Dopamine Reuptake Inhibitor (SNDRI) . It primarily targets three neurotransmitters in the brain:
- Serotonin
- Norepinephrine
- Dopamine
By inhibiting the reuptake of these neurotransmitters, tesofensine increases their availability in the synaptic cleft, which influences appetite regulation and mood. This mechanism is hypothesized to contribute to weight loss by promoting satiety and reducing food intake.
Pharmacokinetics
The pharmacokinetics of this compound reveal important characteristics:
- Half-life : Approximately 220 hours (9 days), allowing for sustained effects.
- Metabolism : Primarily metabolized by cytochrome P4503A4 (CYP3A4) to its active metabolite M1.
- Dosage : Effective doses in clinical studies range from 0.25 mg to 1.0 mg once daily.
Weight Loss Efficacy
Clinical trials have demonstrated significant weight loss associated with tesofensine treatment:
Study | Dosage | Mean Weight Loss (kg) | Duration |
---|---|---|---|
TIPO-1 | 0.5 mg | 13-14 kg | 48 weeks |
Phase II Trial | 0.5 mg | 9.2 kg | 24 weeks |
Phase II Trial | 1.0 mg | 10.6 kg | 24 weeks |
In a randomized, double-blind trial involving 203 obese patients, those treated with tesofensine experienced a mean weight loss significantly greater than those on placebo (p<0.0001) . The most common adverse effects reported included dry mouth, nausea, and insomnia.
Case Studies
- TIPO-4 Study : This open-label extension trial involved patients who had previously completed a 24-week trial. Those who continued with tesofensine maintained significant weight loss compared to those who received placebo during the initial phase .
- Prader-Willi Syndrome : A study indicated that tesofensine could reduce hyperphagia and body weight in patients with Prader-Willi syndrome at lower doses (≤0.250 mg) .
Biochemical Pathways
This compound's action involves several biochemical pathways:
- Neurotransmitter Modulation : It enhances the activity of serotonin, norepinephrine, and dopamine receptors.
- Appetite Regulation : By modulating these neurotransmitters, tesofensine influences hunger and satiety signals in the hypothalamus.
Q & A
Basic Research Questions
Q. What are the primary mechanisms of action of Tesofensine citrate in neurotransmitter systems, and how are these evaluated experimentally?
this compound inhibits the presynaptic reuptake of noradrenaline, dopamine, and serotonin, indirectly stimulating the cholinergic system . Methodologically, in vitro assays (e.g., radioligand binding studies) quantify inhibition potency, while in vivo animal models (e.g., Morris water maze for memory) assess cognitive effects. Researchers should validate findings using microdialysis to measure extracellular neurotransmitter levels in target brain regions .
Q. Which preclinical models are most relevant for evaluating this compound’s cognitive and metabolic effects?
- Cognitive effects : Rodent models of Alzheimer’s disease (e.g., transgenic APP/PS1 mice) or age-related cognitive decline, using behavioral tests like novel object recognition .
- Metabolic effects : Diet-induced obesity (DIO) mice or Zucker fatty rats, with endpoints including body weight, adiposity, and serum leptin levels. Ensure dose selection aligns with human pharmacokinetic profiles (e.g., half-life >200 hours) to avoid overestimation .
Q. How do pharmacokinetic properties of this compound influence clinical trial design?
Tesofensine’s long half-life (>200 hours) and CYP3A4-dependent metabolism necessitate staggered dosing (e.g., weekly administration) to avoid accumulation. Steady-state trough concentrations (~1/3 of peak levels) should guide safety monitoring for CNS or cardiovascular side effects . Bioequivalence studies comparing parent drug and metabolite M1 (5x lower potency) are critical for interpreting efficacy .
Advanced Research Questions
Q. How can researchers reconcile contradictory findings between Tesofensine’s preclinical cognitive benefits and failed Phase IIb clinical trials in Alzheimer’s disease?
The Phase IIb trial’s lack of efficacy may stem from:
- Population heterogeneity : Subgroup analysis of mild vs. moderate Alzheimer’s patients using biomarkers (e.g., CSF Aβ42).
- Dose limitations : Preclinical models used higher doses relative to human equivalents; re-evaluate safety-to-efficacy ratios .
- Outcome measures : Incorporate functional MRI or tau PET imaging to assess target engagement beyond cognitive scales .
Q. What methodological considerations are critical when designing long-term studies on this compound’s metabolic effects?
- Control groups : Use placebo and active comparators (e.g., GLP-1 agonists) to contextualize weight loss efficacy.
- Adherence monitoring : Leverage pharmacokinetic data (e.g., metabolite ratios) to verify compliance .
- Safety endpoints : Prioritize cardiovascular monitoring (e.g., heart rate, blood pressure) due to noradrenergic effects .
Q. What strategies are recommended for analyzing synergistic effects of this compound with other weight loss agents?
- Experimental design : Use factorial designs (e.g., Tesofensine + metformin vs. monotherapy) to assess additive effects.
- Data analysis : Apply isobolographic analysis to distinguish synergy from mere additive interactions.
- Mechanistic validation : Combine microdialysis (neurotransmitters) with indirect calorimetry (energy expenditure) .
Q. Data Contradiction and Reproducibility
Q. How should researchers address discrepancies in this compound’s reported efficacy across animal strains?
- Strain-specific protocols : Standardize diets and environmental conditions (e.g., circadian timing) in DIO models.
- Meta-analysis : Pool data from multiple studies to identify confounding variables (e.g., sex differences in metabolic response) .
Q. What statistical approaches mitigate bias in this compound’s clinical trial data interpretation?
- Pre-specified endpoints : Avoid post-hoc analyses unless hypothesis-driven.
- Bayesian methods : Incorporate prior preclinical data to refine effect size estimates.
- Sensitivity analysis : Test robustness against missing data (e.g., dropout rates in long-term trials) .
Q. Tables: Key Pharmacokinetic and Clinical Trial Data
Parameter | Value | Source |
---|---|---|
Half-life (Tesofensine) | >200 hours | |
Major Metabolite | M1 (CYP3A4-derived) | |
M1 Relative Potency | 5x lower vs. parent compound | |
Phase IIb Trial Outcome | No significant cognitive improvement |
Properties
IUPAC Name |
(1R,2R,3S,5S)-3-(3,4-dichlorophenyl)-2-(ethoxymethyl)-8-methyl-8-azabicyclo[3.2.1]octane;2-hydroxypropane-1,2,3-tricarboxylic acid | |
---|---|---|
Source | PubChem | |
URL | https://pubchem.ncbi.nlm.nih.gov | |
Description | Data deposited in or computed by PubChem | |
InChI |
InChI=1S/C17H23Cl2NO.C6H8O7/c1-3-21-10-14-13(9-12-5-7-17(14)20(12)2)11-4-6-15(18)16(19)8-11;7-3(8)1-6(13,5(11)12)2-4(9)10/h4,6,8,12-14,17H,3,5,7,9-10H2,1-2H3;13H,1-2H2,(H,7,8)(H,9,10)(H,11,12)/t12-,13+,14+,17+;/m0./s1 | |
Source | PubChem | |
URL | https://pubchem.ncbi.nlm.nih.gov | |
Description | Data deposited in or computed by PubChem | |
InChI Key |
ZIVJUFNVDKADJT-BEDQTAKTSA-N | |
Source | PubChem | |
URL | https://pubchem.ncbi.nlm.nih.gov | |
Description | Data deposited in or computed by PubChem | |
Canonical SMILES |
CCOCC1C2CCC(N2C)CC1C3=CC(=C(C=C3)Cl)Cl.C(C(=O)O)C(CC(=O)O)(C(=O)O)O | |
Source | PubChem | |
URL | https://pubchem.ncbi.nlm.nih.gov | |
Description | Data deposited in or computed by PubChem | |
Isomeric SMILES |
CCOC[C@H]1[C@H]2CC[C@H](N2C)C[C@@H]1C3=CC(=C(C=C3)Cl)Cl.C(C(=O)O)C(CC(=O)O)(C(=O)O)O | |
Source | PubChem | |
URL | https://pubchem.ncbi.nlm.nih.gov | |
Description | Data deposited in or computed by PubChem | |
Molecular Formula |
C23H31Cl2NO8 | |
Source | PubChem | |
URL | https://pubchem.ncbi.nlm.nih.gov | |
Description | Data deposited in or computed by PubChem | |
DSSTOX Substance ID |
DTXSID10235407 | |
Record name | Tesofensine citrate | |
Source | EPA DSSTox | |
URL | https://comptox.epa.gov/dashboard/DTXSID10235407 | |
Description | DSSTox provides a high quality public chemistry resource for supporting improved predictive toxicology. | |
Molecular Weight |
520.4 g/mol | |
Source | PubChem | |
URL | https://pubchem.ncbi.nlm.nih.gov | |
Description | Data deposited in or computed by PubChem | |
CAS No. |
195875-86-6, 861205-83-6 | |
Record name | 8-Azabicyclo[3.2.1]octane, 3-(3,4-dichlorophenyl)-2-(ethoxymethyl)-8-methyl-, (1R,2R,3S,5S)-, 2-hydroxy-1,2,3-propanetricarboxylate (1:?) | |
Source | CAS Common Chemistry | |
URL | https://commonchemistry.cas.org/detail?cas_rn=195875-86-6 | |
Description | CAS Common Chemistry is an open community resource for accessing chemical information. Nearly 500,000 chemical substances from CAS REGISTRY cover areas of community interest, including common and frequently regulated chemicals, and those relevant to high school and undergraduate chemistry classes. This chemical information, curated by our expert scientists, is provided in alignment with our mission as a division of the American Chemical Society. | |
Explanation | The data from CAS Common Chemistry is provided under a CC-BY-NC 4.0 license, unless otherwise stated. | |
Record name | Tesofensine citrate | |
Source | ChemIDplus | |
URL | https://pubchem.ncbi.nlm.nih.gov/substance/?source=chemidplus&sourceid=0861205836 | |
Description | ChemIDplus is a free, web search system that provides access to the structure and nomenclature authority files used for the identification of chemical substances cited in National Library of Medicine (NLM) databases, including the TOXNET system. | |
Record name | Tesofensine citrate | |
Source | EPA DSSTox | |
URL | https://comptox.epa.gov/dashboard/DTXSID10235407 | |
Description | DSSTox provides a high quality public chemistry resource for supporting improved predictive toxicology. | |
Record name | TESOFENSINE CITRATE | |
Source | FDA Global Substance Registration System (GSRS) | |
URL | https://gsrs.ncats.nih.gov/ginas/app/beta/substances/3R9T98ZB7U | |
Description | The FDA Global Substance Registration System (GSRS) enables the efficient and accurate exchange of information on what substances are in regulated products. Instead of relying on names, which vary across regulatory domains, countries, and regions, the GSRS knowledge base makes it possible for substances to be defined by standardized, scientific descriptions. | |
Explanation | Unless otherwise noted, the contents of the FDA website (www.fda.gov), both text and graphics, are not copyrighted. They are in the public domain and may be republished, reprinted and otherwise used freely by anyone without the need to obtain permission from FDA. Credit to the U.S. Food and Drug Administration as the source is appreciated but not required. | |
Retrosynthesis Analysis
AI-Powered Synthesis Planning: Our tool employs the Template_relevance Pistachio, Template_relevance Bkms_metabolic, Template_relevance Pistachio_ringbreaker, Template_relevance Reaxys, Template_relevance Reaxys_biocatalysis model, leveraging a vast database of chemical reactions to predict feasible synthetic routes.
One-Step Synthesis Focus: Specifically designed for one-step synthesis, it provides concise and direct routes for your target compounds, streamlining the synthesis process.
Accurate Predictions: Utilizing the extensive PISTACHIO, BKMS_METABOLIC, PISTACHIO_RINGBREAKER, REAXYS, REAXYS_BIOCATALYSIS database, our tool offers high-accuracy predictions, reflecting the latest in chemical research and data.
Strategy Settings
Precursor scoring | Relevance Heuristic |
---|---|
Min. plausibility | 0.01 |
Model | Template_relevance |
Template Set | Pistachio/Bkms_metabolic/Pistachio_ringbreaker/Reaxys/Reaxys_biocatalysis |
Top-N result to add to graph | 6 |
Feasible Synthetic Routes
Disclaimer and Information on In-Vitro Research Products
Please be aware that all articles and product information presented on BenchChem are intended solely for informational purposes. The products available for purchase on BenchChem are specifically designed for in-vitro studies, which are conducted outside of living organisms. In-vitro studies, derived from the Latin term "in glass," involve experiments performed in controlled laboratory settings using cells or tissues. It is important to note that these products are not categorized as medicines or drugs, and they have not received approval from the FDA for the prevention, treatment, or cure of any medical condition, ailment, or disease. We must emphasize that any form of bodily introduction of these products into humans or animals is strictly prohibited by law. It is essential to adhere to these guidelines to ensure compliance with legal and ethical standards in research and experimentation.