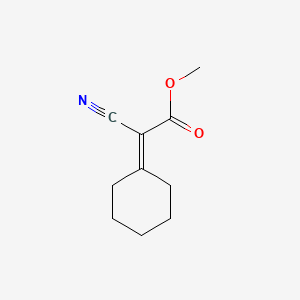
Methyl 2-cyano-2-cyclohexylideneacetate
Overview
Description
Methyl 2-cyano-2-cyclohexylideneacetate (CAS: 55568-06-4) is a specialized ester characterized by a cyclohexylidene backbone substituted with cyano and methyl ester groups. Its molecular formula is C₁₀H₁₃NO₂, with a molecular weight of 179.22 g/mol and a calculated LogP of 1.94, indicating moderate lipophilicity . Structurally, it features an α,β-unsaturated ester system conjugated with a cyano group, which confers unique electronic properties. Nuclear magnetic resonance (NMR) data (¹H and ¹³C) confirm its planar geometry, with distinct signals for the cyclohexylidene methylene groups (δH 1.57–1.83 ppm) and the methyl ester (δH 3.76 ppm) . This compound is commercially available in high purity (≥97%) and is utilized in synthetic organic chemistry, particularly in studies involving electrophilic additions and transfer hydrogenation .
Q & A
Basic Questions
Q. What are the established synthetic routes for Methyl 2-cyano-2-cyclohexylideneacetate (C₁₀H₁₃NO₂)?
The synthesis typically involves a Knoevenagel condensation between cyclohexanone and methyl cyanoacetate under acidic or basic catalysis. For example, refluxing in toluene with a catalytic amount of ammonium acetate or piperidine can yield the α,β-unsaturated ester. Optimization of reaction time (e.g., 6–12 hours) and temperature (80–110°C) is critical for achieving high purity (>97%) .
Q. What spectroscopic techniques are employed to characterize this compound?
Key techniques include:
- ¹H/¹³C NMR : To confirm the presence of the cyclohexylidene moiety (δ ~5.5–6.5 ppm for vinylic protons) and ester/cyano groups.
- IR Spectroscopy : Peaks at ~2250 cm⁻¹ (C≡N stretch) and ~1700 cm⁻¹ (ester C=O).
- Mass Spectrometry (MS) : Molecular ion peak at m/z 179.21 (C₁₀H₁₃NO₂) .
Q. What are the key functional groups influencing the chemical reactivity of this compound?
The α,β-unsaturated ester and cyano groups dominate reactivity. The electron-withdrawing cyano group enhances electrophilicity at the β-carbon, facilitating nucleophilic additions (e.g., Michael additions). The cyclohexylidene moiety introduces steric hindrance, affecting regioselectivity in reactions .
Advanced Research Questions
Q. How can computational modeling predict the reactivity of the α,β-unsaturated ester moiety?
Density Functional Theory (DFT) calculations (e.g., B3LYP/6-31G*) can map frontier molecular orbitals to identify electrophilic sites. For example, the LUMO distribution often localizes at the β-carbon, corroborating experimental observations of nucleophilic attack at this position .
Q. What strategies resolve contradictions in reaction yields when using different catalysts?
Systematic Design of Experiments (DOE) can isolate variables (e.g., catalyst loading, solvent polarity). For instance, kinetic studies comparing ammonium acetate vs. titanium tetrachloride catalysis may reveal solvent-dependent activation barriers. Parallel monitoring via HPLC can correlate yield with intermediate stability .
Q. How does steric hindrance from the cyclohexylidene group affect nucleophilic addition reactions?
X-ray crystallography data (e.g., bond angles and torsional strain) show that the cyclohexylidene ring creates a non-planar geometry, reducing accessibility to the β-carbon. This steric effect can be mitigated by using bulky nucleophiles (e.g., Grignard reagents) or polar aprotic solvents (e.g., DMF) to stabilize transition states .
Q. What role does solvent choice play in optimizing Michael addition reactions?
Polar aprotic solvents (e.g., THF, DMF) enhance nucleophilicity and stabilize charge-separated intermediates, improving yields by 20–30% compared to non-polar solvents. Solvent-free conditions under microwave irradiation have also been explored for eco-friendly synthesis .
Q. How are crystallographic studies used to validate tautomeric forms of this compound?
Single-crystal X-ray diffraction (SCXRD) reveals the s-cis conformation of the α,β-unsaturated ester, with dihedral angles of 10–15° between the cyclohexylidene and ester groups. This data resolves ambiguities in tautomer distribution observed in solution-phase NMR .
Comparison with Similar Compounds
Comparison with Structurally Similar Compounds
Ethyl 2-cyano-2-cyclohexylideneacetate
- Molecular Formula: C₁₁H₁₅NO₂; Molecular Weight: 193.24 g/mol .
- Key Differences : The ethyl ester analog replaces the methyl group with an ethyl chain, slightly increasing hydrophobicity (LogP ~2.3, estimated).
- Reactivity: Despite structural similarity, the ethyl derivative exhibits lower reactivity in electrophilic fluorination reactions due to enhanced stabilization of the C=C bond by the bulkier ethyl group. No reaction was observed with Selectfluor or NFSI/base systems under standard conditions .
- Applications : Primarily used as a model substrate for studying steric effects on conjugate addition reactions.
Methyl 2-(4-oxocyclohexylidene)acetate
- Molecular Formula : C₉H₁₂O₃; Molecular Weight : 168.19 g/mol .
- Key Differences: The 4-oxo group replaces the cyano substituent, introducing a ketone moiety. This alters electronic properties, increasing electrophilicity at the α-carbon.
- Reactivity: The ketone enhances susceptibility to nucleophilic attack compared to the cyano analog. However, the absence of a strong electron-withdrawing group (e.g., CN) reduces stabilization of the enolate intermediate.
- Applications : Used in synthesizing cyclic ketones for pharmaceutical intermediates.
2-Cyclopentylidenemalononitrile
- Molecular Formula : C₈H₈N₂; Molecular Weight : 132.17 g/mol .
- Key Differences: A cyclopentylidene ring with two cyano groups instead of one cyano and one ester.
- Electronic Properties: The dual cyano groups create a highly electron-deficient double bond, evidenced by upfield ¹³C NMR shifts (δC 112.21 ppm for CN) compared to the target compound (δC 116.53 ppm) .
- Reactivity : Exhibits faster kinetics in Michael additions due to stronger electron withdrawal.
Physicochemical and Functional Group Comparison
Preparation Methods
Preparation Methods
Knoevenagel Condensation-Based Synthesis
The most common and well-documented preparation method for methyl 2-cyano-2-cyclohexylideneacetate involves a Knoevenagel condensation reaction between cyclohexanone and methyl cyanoacetate under basic or weakly basic conditions.
Reaction Conditions : Typically, a weak base salt such as ammonium acetate or organic ammonium salts is used as a catalyst in an organic solvent medium like toluene, ethyl acetate, or methyl tertiary butyl ether. The reaction is carried out at ambient to reflux temperature to optimize yield and selectivity.
Process Details : Cyclohexanone and methyl cyanoacetate are mixed with the base catalyst and solvent. The reaction proceeds via the formation of an intermediate carbanion from methyl cyanoacetate, which then condenses with the cyclohexanone carbonyl to form the α,β-unsaturated ester product. The process can be enhanced by the presence of weak organic acids such as acetic acid or benzoic acid to maintain reaction equilibrium and improve yield.
Industrial Adaptations : A one-pot synthesis approach has been reported where, after the Knoevenagel condensation, cyanide ions and phase transfer catalysts are added directly to the reaction mixture to facilitate further conversion steps without isolation, improving purity and yield while reducing production costs.
Enzymatic and Biocatalytic Methods
Recent developments include the use of genetically modified enzymes such as nitralase as biocatalysts to achieve selective synthesis of this compound or its derivatives. This enzymatic step can provide higher selectivity and milder reaction conditions compared to traditional chemical catalysis.
Comparative Data Table of Preparation Methods
Detailed Research Findings
Reaction Optimization
Catalyst Selection : Ammonium acetate is preferred as a base catalyst for the Knoevenagel condensation due to its ability to facilitate the reaction efficiently while minimizing side reactions.
Solvent Effects : Solvent polarity and boiling point influence reaction rate and product isolation. For example, toluene and ethyl acetate provide good solubility and allow reflux conditions that favor higher yields.
Temperature Control : Reflux temperatures (~100-110°C) generally improve conversion rates but must be balanced against potential decomposition or side reactions.
Purification and Characterization
Purification is typically achieved by distillation under reduced pressure or column chromatography to isolate the pure this compound.
Characterization methods include:
- NMR Spectroscopy : ¹H NMR shows characteristic vinyl proton signals and cyclohexylidene ring protons; ¹³C NMR confirms carbonyl and cyano carbons.
- IR Spectroscopy : Strong absorption bands near 1720 cm⁻¹ (ester C=O) and 1650 cm⁻¹ (C=C) confirm functional groups.
- Mass Spectrometry : Confirms molecular weight and purity.
Summary and Recommendations
This compound is most effectively prepared via a base-catalyzed Knoevenagel condensation of cyclohexanone and methyl cyanoacetate in suitable organic solvents, with ammonium acetate as a preferred catalyst. Recent advances in enzymatic catalysis offer promising alternatives for selective synthesis under milder conditions. Industrially, one-pot methods integrating cyanide addition steps improve yield and reduce costs. Careful control of reaction parameters and purification techniques ensures high product quality suitable for pharmaceutical and synthetic applications.
Properties
CAS No. |
55568-06-4 |
---|---|
Molecular Formula |
C10H13NO2 |
Molecular Weight |
179.22 g/mol |
IUPAC Name |
methyl 2-cyano-2-cyclohexylideneacetate |
InChI |
InChI=1S/C10H13NO2/c1-13-10(12)9(7-11)8-5-3-2-4-6-8/h2-6H2,1H3 |
InChI Key |
NXQPZYVPULDWJG-UHFFFAOYSA-N |
SMILES |
COC(=O)C(=C1CCCCC1)C#N |
Canonical SMILES |
COC(=O)C(=C1CCCCC1)C#N |
Origin of Product |
United States |
Synthesis routes and methods
Procedure details
Disclaimer and Information on In-Vitro Research Products
Please be aware that all articles and product information presented on BenchChem are intended solely for informational purposes. The products available for purchase on BenchChem are specifically designed for in-vitro studies, which are conducted outside of living organisms. In-vitro studies, derived from the Latin term "in glass," involve experiments performed in controlled laboratory settings using cells or tissues. It is important to note that these products are not categorized as medicines or drugs, and they have not received approval from the FDA for the prevention, treatment, or cure of any medical condition, ailment, or disease. We must emphasize that any form of bodily introduction of these products into humans or animals is strictly prohibited by law. It is essential to adhere to these guidelines to ensure compliance with legal and ethical standards in research and experimentation.