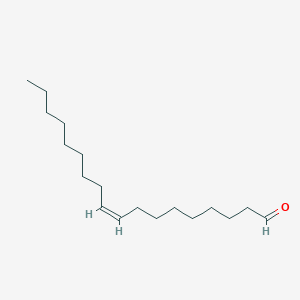
cis-9-Octadecenal
Overview
Description
“Cis-9-Octadecenal” is a chemical compound with the formula C18H34O . It is also known by other names such as Olealdehyde, Oleylaldehyde, and Z-9-Octadecenal .
Molecular Structure Analysis
The molecular structure of “cis-9-Octadecenal” consists of 18 carbon atoms, 34 hydrogen atoms, and 1 oxygen atom . The IUPAC Standard InChIKey for this compound is ZENZJGDPWWLORF-KTKRTIGZSA-N .Physical And Chemical Properties Analysis
“Cis-9-Octadecenal” has a molecular weight of 266.4620 . More specific physical and chemical properties are not provided in the search results.Scientific Research Applications
Tribological Properties :
- Oleic acid-modified TiO2 nanoparticles demonstrate significant antiwear and friction reduction properties, as well as load-carrying capacity, making them beneficial in tribological applications (Gao, Chen, Oli, Zhang, & Xue, 2002).
Chemical Isomerization :
- Isomerization studies involving cis-9-Octadecenal show the transformation of its cis form to a trans form, revealing insights into the behavior of fatty acid molecules under various conditions (Vigier, Barrault, & Pouilloux, 2009).
Absorption and Environmental Applications :
- Cross-linked co-polymer gels of cis-9-Octadecenal exhibit high swelling capacities in various organic solvents and oils, suggesting potential applications in environmental cleanup and water purification (Dutta, Gogoi, Dass, & Sarma, 2013).
Pharmacological Effects :
- Oleamide, a derivative of cis-9-Octadecenal, has been studied for its anxiolytic-like effects in mice, indicating potential applications in the development of anti-anxiety therapies (Wei, Yang, Dong, & Wu, 2007).
Catalysis in Food Industry :
- Research into the catalytic isomerization of linoleic acid, which is structurally related to cis-9-Octadecenal, shows applications in producing anticarcinogenic food constituents (Bernas et al., 2003).
Anti-Inflammatory Effects :
- Studies on oleamide, a cis-9-Octadecenal derivative, demonstrate its ability to inhibit inflammatory responses in microglial cells, suggesting its therapeutic potential in neuroinflammation-related conditions (Oh et al., 2010).
Safety and Hazards
properties
IUPAC Name |
(Z)-octadec-9-enal | |
---|---|---|
Source | PubChem | |
URL | https://pubchem.ncbi.nlm.nih.gov | |
Description | Data deposited in or computed by PubChem | |
InChI |
InChI=1S/C18H34O/c1-2-3-4-5-6-7-8-9-10-11-12-13-14-15-16-17-18-19/h9-10,18H,2-8,11-17H2,1H3/b10-9- | |
Source | PubChem | |
URL | https://pubchem.ncbi.nlm.nih.gov | |
Description | Data deposited in or computed by PubChem | |
InChI Key |
ZENZJGDPWWLORF-KTKRTIGZSA-N | |
Source | PubChem | |
URL | https://pubchem.ncbi.nlm.nih.gov | |
Description | Data deposited in or computed by PubChem | |
Canonical SMILES |
CCCCCCCCC=CCCCCCCCC=O | |
Source | PubChem | |
URL | https://pubchem.ncbi.nlm.nih.gov | |
Description | Data deposited in or computed by PubChem | |
Isomeric SMILES |
CCCCCCCC/C=C\CCCCCCCC=O | |
Source | PubChem | |
URL | https://pubchem.ncbi.nlm.nih.gov | |
Description | Data deposited in or computed by PubChem | |
Molecular Formula |
C18H34O | |
Source | PubChem | |
URL | https://pubchem.ncbi.nlm.nih.gov | |
Description | Data deposited in or computed by PubChem | |
DSSTOX Substance ID |
DTXSID101016447 | |
Record name | 9-Octadecenal, (9Z)- | |
Source | EPA DSSTox | |
URL | https://comptox.epa.gov/dashboard/DTXSID101016447 | |
Description | DSSTox provides a high quality public chemistry resource for supporting improved predictive toxicology. | |
Molecular Weight |
266.5 g/mol | |
Source | PubChem | |
URL | https://pubchem.ncbi.nlm.nih.gov | |
Description | Data deposited in or computed by PubChem | |
Product Name |
cis-9-Octadecenal | |
CAS RN |
2423-10-1 | |
Record name | 9-Octadecenal, (9Z)- | |
Source | ChemIDplus | |
URL | https://pubchem.ncbi.nlm.nih.gov/substance/?source=chemidplus&sourceid=0002423101 | |
Description | ChemIDplus is a free, web search system that provides access to the structure and nomenclature authority files used for the identification of chemical substances cited in National Library of Medicine (NLM) databases, including the TOXNET system. | |
Record name | 9-Octadecenal, (9Z)- | |
Source | EPA DSSTox | |
URL | https://comptox.epa.gov/dashboard/DTXSID101016447 | |
Description | DSSTox provides a high quality public chemistry resource for supporting improved predictive toxicology. | |
Record name | 9-OCTADECENAL, (9Z)- | |
Source | FDA Global Substance Registration System (GSRS) | |
URL | https://gsrs.ncats.nih.gov/ginas/app/beta/substances/753Z607472 | |
Description | The FDA Global Substance Registration System (GSRS) enables the efficient and accurate exchange of information on what substances are in regulated products. Instead of relying on names, which vary across regulatory domains, countries, and regions, the GSRS knowledge base makes it possible for substances to be defined by standardized, scientific descriptions. | |
Explanation | Unless otherwise noted, the contents of the FDA website (www.fda.gov), both text and graphics, are not copyrighted. They are in the public domain and may be republished, reprinted and otherwise used freely by anyone without the need to obtain permission from FDA. Credit to the U.S. Food and Drug Administration as the source is appreciated but not required. | |
Synthesis routes and methods
Procedure details
Retrosynthesis Analysis
AI-Powered Synthesis Planning: Our tool employs the Template_relevance Pistachio, Template_relevance Bkms_metabolic, Template_relevance Pistachio_ringbreaker, Template_relevance Reaxys, Template_relevance Reaxys_biocatalysis model, leveraging a vast database of chemical reactions to predict feasible synthetic routes.
One-Step Synthesis Focus: Specifically designed for one-step synthesis, it provides concise and direct routes for your target compounds, streamlining the synthesis process.
Accurate Predictions: Utilizing the extensive PISTACHIO, BKMS_METABOLIC, PISTACHIO_RINGBREAKER, REAXYS, REAXYS_BIOCATALYSIS database, our tool offers high-accuracy predictions, reflecting the latest in chemical research and data.
Strategy Settings
Precursor scoring | Relevance Heuristic |
---|---|
Min. plausibility | 0.01 |
Model | Template_relevance |
Template Set | Pistachio/Bkms_metabolic/Pistachio_ringbreaker/Reaxys/Reaxys_biocatalysis |
Top-N result to add to graph | 6 |
Feasible Synthetic Routes
Disclaimer and Information on In-Vitro Research Products
Please be aware that all articles and product information presented on BenchChem are intended solely for informational purposes. The products available for purchase on BenchChem are specifically designed for in-vitro studies, which are conducted outside of living organisms. In-vitro studies, derived from the Latin term "in glass," involve experiments performed in controlled laboratory settings using cells or tissues. It is important to note that these products are not categorized as medicines or drugs, and they have not received approval from the FDA for the prevention, treatment, or cure of any medical condition, ailment, or disease. We must emphasize that any form of bodily introduction of these products into humans or animals is strictly prohibited by law. It is essential to adhere to these guidelines to ensure compliance with legal and ethical standards in research and experimentation.