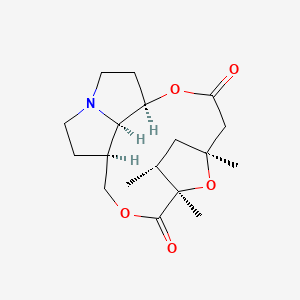
Nemorensine
Overview
Description
Nemorensine is a macrolide . Its molecular formula is C18H27NO5 . It has an average mass of 337.411 Da and a monoisotopic mass of 337.188934 Da .
Synthesis Analysis
The synthesis of this compound has been described in various studies. For instance, the synthesis of (+)-nemorensic acid, a component of this compound, was achieved starting from a commercially available furoic acid . The process involved nine steps and resulted in a 32% overall yield . Key steps in the sequence included a chiral auxiliary controlled, stereoselective Birch reduction of 3-methyl-2-furoic acid, and the stereoselective reaction of an oxonium ion generated within a tetrahydrofuran ring .
Molecular Structure Analysis
This compound has 5 of 6 defined stereocentres . Its structure is also available as a 2D Mol file .
Chemical Reactions Analysis
Several chemical reactions are involved in the synthesis of this compound. These include the Birch reduction of 3-methyl-2-furoic acid and the reaction of an oxonium ion generated within a tetrahydrofuran ring . Other reactions such as oxidative cyclization based on reversing the polarity of enol ethers and ketene dithioacetals have also been reported .
Physical and Chemical Properties Analysis
This compound is a powder . Its molecular weight is 337.4107 .
Scientific Research Applications
Synthesis and Chemical Studies
- Synthesis of Nemorensic Acid: A study by Donohoe, Guillermin, and Walter (2002) described the synthesis of (+)-nemorensic acid from commercially available furoic acid. This research is significant for understanding the chemical properties and potential applications of nemorensine, even though the complete synthesis of this compound was not achieved due to the low nucleophilicity of platynecine (Donohoe, Guillermin, & Walter, 2002).
Neuroendocrine Neoplasms and Digestive Tract
- Neuroendocrine Neoplasms (NENs) and Digestive Tract: Research by Pobłocki et al. (2020) explored the diagnosis, treatment, and nutrition of neuroendocrine neoplasms (NENs), primarily of the digestive and respiratory tract. Although this study does not directly reference this compound, the understanding of NENs, their treatment, and biochemical properties may provide insights for potential future applications of this compound in these areas (Pobłocki et al., 2020).
Neurotransmitter Modulation
- Modulation of Synaptic Transmission: A 2007 study by Zhang and Mifflin investigated the role of α1-adrenoreceptors in synaptic transmission of peripheral chemoreceptor inputs. This research might be relevant in the context of this compound's potential interaction with neurotransmitter systems (Zhang & Mifflin, 2007).
Neuroendocrine Neoplasms Classification
- Neuroendocrine Neoplasms Classification: A 2018 study proposed a common classification framework for neuroendocrine neoplasms at various anatomical locations. This framework, while not directly involving this compound, is crucial for understanding the diseases that this compound could potentially target or affect (Rindi et al., 2018).
Role in Neuroendocrine Neoplasms
- Role in Neuroendocrine Neoplasms: Vitale et al. (2021) reviewed the role of the fibroblast growth factor (FGF) system in the development and progression of neuroendocrine neoplasms. Understanding the FGF system's role can provide insights into the potential impact of this compound in similar pathways or neoplasms (Vitale et al., 2021).
Safety and Hazards
Properties
IUPAC Name |
(1R,5S,7R,8R,12S,18R)-5,7,8-trimethyl-2,10,19-trioxa-15-azatetracyclo[10.5.1.15,8.015,18]nonadecane-3,9-dione | |
---|---|---|
Source | PubChem | |
URL | https://pubchem.ncbi.nlm.nih.gov | |
Description | Data deposited in or computed by PubChem | |
InChI |
InChI=1S/C18H27NO5/c1-11-8-17(2)9-14(20)23-13-5-7-19-6-4-12(15(13)19)10-22-16(21)18(11,3)24-17/h11-13,15H,4-10H2,1-3H3/t11-,12-,13-,15-,17+,18-/m1/s1 | |
Source | PubChem | |
URL | https://pubchem.ncbi.nlm.nih.gov | |
Description | Data deposited in or computed by PubChem | |
InChI Key |
DNEINKNDPRUHLP-WLFFGFHMSA-N | |
Source | PubChem | |
URL | https://pubchem.ncbi.nlm.nih.gov | |
Description | Data deposited in or computed by PubChem | |
Canonical SMILES |
CC1CC2(CC(=O)OC3CCN4C3C(CC4)COC(=O)C1(O2)C)C | |
Source | PubChem | |
URL | https://pubchem.ncbi.nlm.nih.gov | |
Description | Data deposited in or computed by PubChem | |
Isomeric SMILES |
C[C@@H]1C[C@]2(CC(=O)O[C@@H]3CCN4[C@@H]3[C@H](CC4)COC(=O)[C@@]1(O2)C)C | |
Source | PubChem | |
URL | https://pubchem.ncbi.nlm.nih.gov | |
Description | Data deposited in or computed by PubChem | |
Molecular Formula |
C18H27NO5 | |
Source | PubChem | |
URL | https://pubchem.ncbi.nlm.nih.gov | |
Description | Data deposited in or computed by PubChem | |
Molecular Weight |
337.4 g/mol | |
Source | PubChem | |
URL | https://pubchem.ncbi.nlm.nih.gov | |
Description | Data deposited in or computed by PubChem | |
CAS No. |
50906-96-2 | |
Record name | (4S,6R,7R,10aS,15aR,15bR)-Dodecahydro-4,6,7-trimethyl-4,7-epoxy-2H-[1,6]dioxacyclotridecino[2,3,4-gh]pyrrolizine-2,8(3H)-dione | |
Source | CAS Common Chemistry | |
URL | https://commonchemistry.cas.org/detail?cas_rn=50906-96-2 | |
Description | CAS Common Chemistry is an open community resource for accessing chemical information. Nearly 500,000 chemical substances from CAS REGISTRY cover areas of community interest, including common and frequently regulated chemicals, and those relevant to high school and undergraduate chemistry classes. This chemical information, curated by our expert scientists, is provided in alignment with our mission as a division of the American Chemical Society. | |
Explanation | The data from CAS Common Chemistry is provided under a CC-BY-NC 4.0 license, unless otherwise stated. | |
Record name | C(16a)-Homo-21-norsenecionan-11,16a-dione, 12,15-epoxy-1,2,15,20-tetrahydro-, (1alpha,12R,15R)- | |
Source | ChemIDplus | |
URL | https://pubchem.ncbi.nlm.nih.gov/substance/?source=chemidplus&sourceid=0050906962 | |
Description | ChemIDplus is a free, web search system that provides access to the structure and nomenclature authority files used for the identification of chemical substances cited in National Library of Medicine (NLM) databases, including the TOXNET system. | |
Retrosynthesis Analysis
AI-Powered Synthesis Planning: Our tool employs the Template_relevance Pistachio, Template_relevance Bkms_metabolic, Template_relevance Pistachio_ringbreaker, Template_relevance Reaxys, Template_relevance Reaxys_biocatalysis model, leveraging a vast database of chemical reactions to predict feasible synthetic routes.
One-Step Synthesis Focus: Specifically designed for one-step synthesis, it provides concise and direct routes for your target compounds, streamlining the synthesis process.
Accurate Predictions: Utilizing the extensive PISTACHIO, BKMS_METABOLIC, PISTACHIO_RINGBREAKER, REAXYS, REAXYS_BIOCATALYSIS database, our tool offers high-accuracy predictions, reflecting the latest in chemical research and data.
Strategy Settings
Precursor scoring | Relevance Heuristic |
---|---|
Min. plausibility | 0.01 |
Model | Template_relevance |
Template Set | Pistachio/Bkms_metabolic/Pistachio_ringbreaker/Reaxys/Reaxys_biocatalysis |
Top-N result to add to graph | 6 |
Feasible Synthetic Routes
Q1: What is Nemorensine and where is it found?
A1: this compound is a pyrrolizidine alkaloid (PA) first isolated from the plant Senecio nemorensis L. [, ]. PAs are a group of naturally occurring toxins produced by various plant species, often as a defense mechanism against herbivores.
Q2: Can you elaborate on the synthetic approaches used to determine the structure of (+)-Nemorensic acid?
A3: Several synthetic routes to (+)-Nemorensic acid have been developed. One approach utilized the readily available monoterpene (-)-carvone and involved a unique fragmentation reaction mediated by samarium iodide to construct the tetrahydrofuran ring present in (+)-Nemorensic acid []. Another strategy employed a stereoselective Birch reduction of 3-methyl-2-furoic acid and took advantage of the reactivity of an oxonium ion within a tetrahydrofuran ring to install the correct stereochemistry [].
Q3: Are there any analytical methods used to quantify this compound?
A4: Yes, a method using flame atomic absorption spectrometry (FAAS) has been developed to indirectly determine the concentration of this compound []. This method involves the formation and extraction of ion pairs between the alkaloid and a bismuth complex, followed by measurement of the metal concentration in the organic phase using FAAS.
Disclaimer and Information on In-Vitro Research Products
Please be aware that all articles and product information presented on BenchChem are intended solely for informational purposes. The products available for purchase on BenchChem are specifically designed for in-vitro studies, which are conducted outside of living organisms. In-vitro studies, derived from the Latin term "in glass," involve experiments performed in controlled laboratory settings using cells or tissues. It is important to note that these products are not categorized as medicines or drugs, and they have not received approval from the FDA for the prevention, treatment, or cure of any medical condition, ailment, or disease. We must emphasize that any form of bodily introduction of these products into humans or animals is strictly prohibited by law. It is essential to adhere to these guidelines to ensure compliance with legal and ethical standards in research and experimentation.