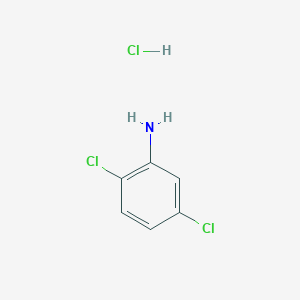
2,5-Dichloroanilinium chloride
Overview
Description
2,5-Dichloroanilinium chloride is an organic compound that has gained significant attention in recent years due to its potential applications in scientific research. It is a salt of 2,5-dichloroaniline, which is a derivative of aniline. This compound has been used in various fields of research, including medicinal chemistry, biochemistry, and environmental science.
Scientific Research Applications
Molecular Structure and Bonding Interactions
Research on 2,5-Dichloroanilinium chloride has contributed significantly to the understanding of molecular structures and bonding interactions. For instance, a study by Gray and Jones (2002) explored the secondary bonding interactions in various dichloroanilinium chlorides, including 2,5-dichloroanilinium chloride. They found that 2,5-dichloroanilinium chloride forms a layer structure directly with rings R36 (12) analogous to those observed in 2-chloroanilinium chloride, highlighting its significance in understanding hydrogen bonding systems and hydrophilic layers in molecular structures (Gray & Jones, 2002).
Optical Properties and Photonics
In photonics, the optical properties of 2,5-Dichloroanilinium chloride have been a subject of interest. Sudharsana et al. (2012) investigated the linear and nonlinear optical properties of 2,5-Dichloroanilinium picrate crystal, which is related to 2,5-Dichloroanilinium chloride. Their research, which included UV-Vis spectroscopy and theoretical studies, contributed to understanding the material's potential in photonics applications (Sudharsana et al., 2012).
Thermal Decomposition and Stability
The thermal properties and stability of dichloroanilinium chlorides, including 2,5-Dichloroanilinium chloride, have been researched. Singh and Srivastava (2004) focused on the kinetics of thermolysis of dichloroanilinium chloride salts. Their study provided insights into the thermal decomposition processes and the stability of these compounds under various conditions (Singh & Srivastava, 2004).
Crystal Structure and Hydrogen Bonding
The study of the crystal structure of 2,5-Dichloroanilinium chloride and its related compounds has been instrumental in understanding hydrogen bonding and molecular interactions. For example, Shakuntala, Foro, and Gowda (2011) examined the crystal of 2,5-Dichloroanilinium 4-chlorobenzenesulfonate, which provides insights into the nature of hydrogen bonds and the structural formation of similar compounds (Shakuntala, Foro, & Gowda, 2011).
Electrochemical Synthesis
The electrochemical synthesis of 2,5-Dichloroaniline, a derivative of 2,5-Dichloroanilinium chloride, has been a topic of interest. Islamgulova et al. (2003) proposed a procedure for electrochemical reduction of 2,5-dichloronitrobenzene to 2,5-dichloroaniline, offering insights into the synthetic processes involving this compound (Islamgulova et al., 2003).
properties
IUPAC Name |
2,5-dichloroaniline;hydrochloride | |
---|---|---|
Source | PubChem | |
URL | https://pubchem.ncbi.nlm.nih.gov | |
Description | Data deposited in or computed by PubChem | |
InChI |
InChI=1S/C6H5Cl2N.ClH/c7-4-1-2-5(8)6(9)3-4;/h1-3H,9H2;1H | |
Source | PubChem | |
URL | https://pubchem.ncbi.nlm.nih.gov | |
Description | Data deposited in or computed by PubChem | |
InChI Key |
ZALVJCYWUBQENP-UHFFFAOYSA-N | |
Source | PubChem | |
URL | https://pubchem.ncbi.nlm.nih.gov | |
Description | Data deposited in or computed by PubChem | |
Canonical SMILES |
C1=CC(=C(C=C1Cl)N)Cl.Cl | |
Source | PubChem | |
URL | https://pubchem.ncbi.nlm.nih.gov | |
Description | Data deposited in or computed by PubChem | |
Molecular Formula |
C6H6Cl3N | |
Source | PubChem | |
URL | https://pubchem.ncbi.nlm.nih.gov | |
Description | Data deposited in or computed by PubChem | |
DSSTOX Substance ID |
DTXSID80955281 | |
Record name | 2,5-Dichloroaniline--hydrogen chloride (1/1) | |
Source | EPA DSSTox | |
URL | https://comptox.epa.gov/dashboard/DTXSID80955281 | |
Description | DSSTox provides a high quality public chemistry resource for supporting improved predictive toxicology. | |
Molecular Weight |
198.5 g/mol | |
Source | PubChem | |
URL | https://pubchem.ncbi.nlm.nih.gov | |
Description | Data deposited in or computed by PubChem | |
Product Name |
2,5-Dichloroanilinium chloride | |
CAS RN |
33663-41-1 | |
Record name | Benzenamine, 2,5-dichloro-, hydrochloride (1:1) | |
Source | CAS Common Chemistry | |
URL | https://commonchemistry.cas.org/detail?cas_rn=33663-41-1 | |
Description | CAS Common Chemistry is an open community resource for accessing chemical information. Nearly 500,000 chemical substances from CAS REGISTRY cover areas of community interest, including common and frequently regulated chemicals, and those relevant to high school and undergraduate chemistry classes. This chemical information, curated by our expert scientists, is provided in alignment with our mission as a division of the American Chemical Society. | |
Explanation | The data from CAS Common Chemistry is provided under a CC-BY-NC 4.0 license, unless otherwise stated. | |
Record name | 2,5-Dichloroanilinium chloride | |
Source | ChemIDplus | |
URL | https://pubchem.ncbi.nlm.nih.gov/substance/?source=chemidplus&sourceid=0033663411 | |
Description | ChemIDplus is a free, web search system that provides access to the structure and nomenclature authority files used for the identification of chemical substances cited in National Library of Medicine (NLM) databases, including the TOXNET system. | |
Record name | 2,5-Dichloroaniline--hydrogen chloride (1/1) | |
Source | EPA DSSTox | |
URL | https://comptox.epa.gov/dashboard/DTXSID80955281 | |
Description | DSSTox provides a high quality public chemistry resource for supporting improved predictive toxicology. | |
Record name | 2,5-dichloroanilinium chloride | |
Source | European Chemicals Agency (ECHA) | |
URL | https://echa.europa.eu/substance-information/-/substanceinfo/100.046.913 | |
Description | The European Chemicals Agency (ECHA) is an agency of the European Union which is the driving force among regulatory authorities in implementing the EU's groundbreaking chemicals legislation for the benefit of human health and the environment as well as for innovation and competitiveness. | |
Explanation | Use of the information, documents and data from the ECHA website is subject to the terms and conditions of this Legal Notice, and subject to other binding limitations provided for under applicable law, the information, documents and data made available on the ECHA website may be reproduced, distributed and/or used, totally or in part, for non-commercial purposes provided that ECHA is acknowledged as the source: "Source: European Chemicals Agency, http://echa.europa.eu/". Such acknowledgement must be included in each copy of the material. ECHA permits and encourages organisations and individuals to create links to the ECHA website under the following cumulative conditions: Links can only be made to webpages that provide a link to the Legal Notice page. | |
Synthesis routes and methods
Procedure details
Retrosynthesis Analysis
AI-Powered Synthesis Planning: Our tool employs the Template_relevance Pistachio, Template_relevance Bkms_metabolic, Template_relevance Pistachio_ringbreaker, Template_relevance Reaxys, Template_relevance Reaxys_biocatalysis model, leveraging a vast database of chemical reactions to predict feasible synthetic routes.
One-Step Synthesis Focus: Specifically designed for one-step synthesis, it provides concise and direct routes for your target compounds, streamlining the synthesis process.
Accurate Predictions: Utilizing the extensive PISTACHIO, BKMS_METABOLIC, PISTACHIO_RINGBREAKER, REAXYS, REAXYS_BIOCATALYSIS database, our tool offers high-accuracy predictions, reflecting the latest in chemical research and data.
Strategy Settings
Precursor scoring | Relevance Heuristic |
---|---|
Min. plausibility | 0.01 |
Model | Template_relevance |
Template Set | Pistachio/Bkms_metabolic/Pistachio_ringbreaker/Reaxys/Reaxys_biocatalysis |
Top-N result to add to graph | 6 |
Feasible Synthetic Routes
Disclaimer and Information on In-Vitro Research Products
Please be aware that all articles and product information presented on BenchChem are intended solely for informational purposes. The products available for purchase on BenchChem are specifically designed for in-vitro studies, which are conducted outside of living organisms. In-vitro studies, derived from the Latin term "in glass," involve experiments performed in controlled laboratory settings using cells or tissues. It is important to note that these products are not categorized as medicines or drugs, and they have not received approval from the FDA for the prevention, treatment, or cure of any medical condition, ailment, or disease. We must emphasize that any form of bodily introduction of these products into humans or animals is strictly prohibited by law. It is essential to adhere to these guidelines to ensure compliance with legal and ethical standards in research and experimentation.