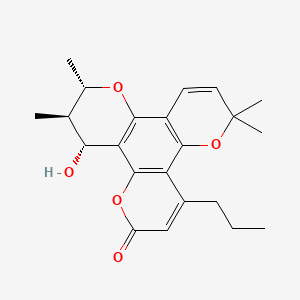
(-)-Calanolide A
Overview
Description
(-)-Calanolide A is a useful research compound. Its molecular formula is C22H26O5 and its molecular weight is 370.4 g/mol. The purity is usually 95%.
The exact mass of the compound this compound is unknown and the complexity rating of the compound is unknown. The compound has been submitted to the National Cancer Institute (NCI) for testing and evaluation and the Cancer Chemotherapy National Service Center (NSC) number is 675449. The storage condition is unknown. Please store according to label instructions upon receipt of goods.
BenchChem offers high-quality this compound suitable for many research applications. Different packaging options are available to accommodate customers' requirements. Please inquire for more information about this compound including the price, delivery time, and more detailed information at info@benchchem.com.
Properties
CAS No. |
163661-45-8 |
---|---|
Molecular Formula |
C22H26O5 |
Molecular Weight |
370.4 g/mol |
IUPAC Name |
(16S,17R,18R)-18-hydroxy-10,10,16,17-tetramethyl-6-propyl-3,9,15-trioxatetracyclo[12.4.0.02,7.08,13]octadeca-1(14),2(7),5,8(13),11-pentaen-4-one |
InChI |
InChI=1S/C22H26O5/c1-6-7-13-10-15(23)26-21-16(13)20-14(8-9-22(4,5)27-20)19-17(21)18(24)11(2)12(3)25-19/h8-12,18,24H,6-7H2,1-5H3/t11-,12-,18+/m0/s1 |
InChI Key |
NIDRYBLTWYFCFV-OAVHHTNSSA-N |
SMILES |
CCCC1=CC(=O)OC2=C1C3=C(C=CC(O3)(C)C)C4=C2C(C(C(O4)C)C)O |
Isomeric SMILES |
CCCC1=CC(=O)OC2=C1C3=C(C=CC(O3)(C)C)C4=C2[C@@H]([C@H]([C@@H](O4)C)C)O |
Canonical SMILES |
CCCC1=CC(=O)OC2=C1C3=C(C=CC(O3)(C)C)C4=C2C(C(C(O4)C)C)O |
Key on ui other cas no. |
163661-45-8 |
Origin of Product |
United States |
Synthesis routes and methods I
Procedure details
Synthesis routes and methods II
Procedure details
Disclaimer and Information on In-Vitro Research Products
Please be aware that all articles and product information presented on BenchChem are intended solely for informational purposes. The products available for purchase on BenchChem are specifically designed for in-vitro studies, which are conducted outside of living organisms. In-vitro studies, derived from the Latin term "in glass," involve experiments performed in controlled laboratory settings using cells or tissues. It is important to note that these products are not categorized as medicines or drugs, and they have not received approval from the FDA for the prevention, treatment, or cure of any medical condition, ailment, or disease. We must emphasize that any form of bodily introduction of these products into humans or animals is strictly prohibited by law. It is essential to adhere to these guidelines to ensure compliance with legal and ethical standards in research and experimentation.