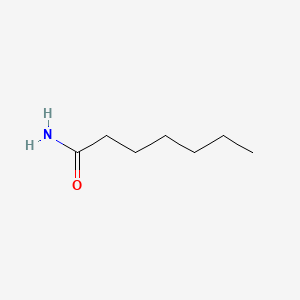
Heptanamide
Overview
Description
Heptanamide, also known as Enanthamide or Enanthic acid amide, is a compound that belongs to the class of organic compounds known as fatty amides . These are carboxylic acid amide derivatives of fatty acids, formed from a fatty acid and an amine . It has a molecular formula of C7H15NO .
Synthesis Analysis
Heptanamide can be synthesized by combining the appropriate amines and acyl chlorides . Another method involves reacting esters with alkali metal amidoboranes .
Molecular Structure Analysis
Heptanamide has a molecular weight of 129.200 Da and a monoisotopic mass of 129.115356 Da . It is composed of 7 carbon atoms, 15 hydrogen atoms, and 1 oxygen atom .
Physical And Chemical Properties Analysis
Heptanamide is a solid substance . Its exact physical and chemical properties are not well-documented in the available resources.
Scientific Research Applications
Pharmacology and Drug Development
Heptanamide is classified as an experimental small molecule under the category of fatty amides . Although specific indications and pharmacodynamics are not detailed, its structure and properties suggest potential applications in the development of therapeutic agents. The interaction of Heptanamide with limonene-1,2-epoxide hydrolase in organisms like Rhodococcus erythropolis could be explored for designing drugs targeting similar enzymes in humans .
Surface Chemistry
The formation and thermal stability of self-assembled monolayers (SAMs) on gold surfaces using derivatives of Heptanamide have been studied . This application is crucial for creating coatings that modify surface properties, such as hydrophobicity or electrical conductivity, which are important in nanotechnology and electronics.
Safety and Hazards
When handling Heptanamide, it’s advised to avoid dust formation, breathing mist, gas, or vapors, and contacting with skin and eye . Use personal protective equipment, wear chemical impermeable gloves, ensure adequate ventilation, remove all sources of ignition, and evacuate personnel to safe areas .
Future Directions
While specific future directions for Heptanamide are not well-documented, the field of therapeutic peptides, which includes compounds like Heptanamide, is advancing with the application of new technologies . Peptide-drug conjugates are being explored for targeted cancer therapy , and therapeutic peptides are being developed for various applications .
Mechanism of Action
Target of Action
Heptanamide, a type of fatty amide , primarily targets the enzyme Limonene-1,2-epoxide hydrolase in Rhodococcus erythropolis . This enzyme plays a crucial role in the metabolism of limonene, a common compound in citrus oils .
Mode of Action
Heptanamide interacts with its target enzyme by serving as a substrate. The enzyme Limonene-1,2-epoxide hydrolase catalyzes the conversion of limonene-1,2-epoxide to limonene-1,2-diol . It can use both the (-) and (+) isomers of limonene-1,2-epoxide as substrates .
Biochemical Pathways
Given its interaction with limonene-1,2-epoxide hydrolase, it’s likely involved in the metabolic pathways of limonene .
Result of Action
The primary result of Heptanamide’s action is the conversion of limonene-1,2-epoxide to limonene-1,2-diol . This conversion is part of the metabolic process of limonene, a compound found in citrus oils .
properties
IUPAC Name |
heptanamide | |
---|---|---|
Source | PubChem | |
URL | https://pubchem.ncbi.nlm.nih.gov | |
Description | Data deposited in or computed by PubChem | |
InChI |
InChI=1S/C7H15NO/c1-2-3-4-5-6-7(8)9/h2-6H2,1H3,(H2,8,9) | |
Source | PubChem | |
URL | https://pubchem.ncbi.nlm.nih.gov | |
Description | Data deposited in or computed by PubChem | |
InChI Key |
AEDIXYWIVPYNBI-UHFFFAOYSA-N | |
Source | PubChem | |
URL | https://pubchem.ncbi.nlm.nih.gov | |
Description | Data deposited in or computed by PubChem | |
Canonical SMILES |
CCCCCCC(=O)N | |
Source | PubChem | |
URL | https://pubchem.ncbi.nlm.nih.gov | |
Description | Data deposited in or computed by PubChem | |
Molecular Formula |
C7H15NO | |
Source | PubChem | |
URL | https://pubchem.ncbi.nlm.nih.gov | |
Description | Data deposited in or computed by PubChem | |
DSSTOX Substance ID |
DTXSID50211886 | |
Record name | Heptanamide | |
Source | EPA DSSTox | |
URL | https://comptox.epa.gov/dashboard/DTXSID50211886 | |
Description | DSSTox provides a high quality public chemistry resource for supporting improved predictive toxicology. | |
Molecular Weight |
129.20 g/mol | |
Source | PubChem | |
URL | https://pubchem.ncbi.nlm.nih.gov | |
Description | Data deposited in or computed by PubChem | |
CAS RN |
628-62-6 | |
Record name | Heptanamide | |
Source | CAS Common Chemistry | |
URL | https://commonchemistry.cas.org/detail?cas_rn=628-62-6 | |
Description | CAS Common Chemistry is an open community resource for accessing chemical information. Nearly 500,000 chemical substances from CAS REGISTRY cover areas of community interest, including common and frequently regulated chemicals, and those relevant to high school and undergraduate chemistry classes. This chemical information, curated by our expert scientists, is provided in alignment with our mission as a division of the American Chemical Society. | |
Explanation | The data from CAS Common Chemistry is provided under a CC-BY-NC 4.0 license, unless otherwise stated. | |
Record name | Heptanamide | |
Source | ChemIDplus | |
URL | https://pubchem.ncbi.nlm.nih.gov/substance/?source=chemidplus&sourceid=0000628626 | |
Description | ChemIDplus is a free, web search system that provides access to the structure and nomenclature authority files used for the identification of chemical substances cited in National Library of Medicine (NLM) databases, including the TOXNET system. | |
Record name | Heptanamide | |
Source | DrugBank | |
URL | https://www.drugbank.ca/drugs/DB02641 | |
Description | The DrugBank database is a unique bioinformatics and cheminformatics resource that combines detailed drug (i.e. chemical, pharmacological and pharmaceutical) data with comprehensive drug target (i.e. sequence, structure, and pathway) information. | |
Explanation | Creative Common's Attribution-NonCommercial 4.0 International License (http://creativecommons.org/licenses/by-nc/4.0/legalcode) | |
Record name | Heptanamide | |
Source | DTP/NCI | |
URL | https://dtp.cancer.gov/dtpstandard/servlet/dwindex?searchtype=NSC&outputformat=html&searchlist=3819 | |
Description | The NCI Development Therapeutics Program (DTP) provides services and resources to the academic and private-sector research communities worldwide to facilitate the discovery and development of new cancer therapeutic agents. | |
Explanation | Unless otherwise indicated, all text within NCI products is free of copyright and may be reused without our permission. Credit the National Cancer Institute as the source. | |
Record name | Heptanamide | |
Source | EPA DSSTox | |
URL | https://comptox.epa.gov/dashboard/DTXSID50211886 | |
Description | DSSTox provides a high quality public chemistry resource for supporting improved predictive toxicology. | |
Record name | HEPTANAMIDE | |
Source | FDA Global Substance Registration System (GSRS) | |
URL | https://gsrs.ncats.nih.gov/ginas/app/beta/substances/6QX6H3415T | |
Description | The FDA Global Substance Registration System (GSRS) enables the efficient and accurate exchange of information on what substances are in regulated products. Instead of relying on names, which vary across regulatory domains, countries, and regions, the GSRS knowledge base makes it possible for substances to be defined by standardized, scientific descriptions. | |
Explanation | Unless otherwise noted, the contents of the FDA website (www.fda.gov), both text and graphics, are not copyrighted. They are in the public domain and may be republished, reprinted and otherwise used freely by anyone without the need to obtain permission from FDA. Credit to the U.S. Food and Drug Administration as the source is appreciated but not required. | |
Retrosynthesis Analysis
AI-Powered Synthesis Planning: Our tool employs the Template_relevance Pistachio, Template_relevance Bkms_metabolic, Template_relevance Pistachio_ringbreaker, Template_relevance Reaxys, Template_relevance Reaxys_biocatalysis model, leveraging a vast database of chemical reactions to predict feasible synthetic routes.
One-Step Synthesis Focus: Specifically designed for one-step synthesis, it provides concise and direct routes for your target compounds, streamlining the synthesis process.
Accurate Predictions: Utilizing the extensive PISTACHIO, BKMS_METABOLIC, PISTACHIO_RINGBREAKER, REAXYS, REAXYS_BIOCATALYSIS database, our tool offers high-accuracy predictions, reflecting the latest in chemical research and data.
Strategy Settings
Precursor scoring | Relevance Heuristic |
---|---|
Min. plausibility | 0.01 |
Model | Template_relevance |
Template Set | Pistachio/Bkms_metabolic/Pistachio_ringbreaker/Reaxys/Reaxys_biocatalysis |
Top-N result to add to graph | 6 |
Feasible Synthetic Routes
Q & A
Q1: What is the mechanism of action for Heptanamide derivatives as histone deacetylase (HDAC) inhibitors?
A1: While the provided abstracts do not delve into specific mechanistic details for all Heptanamide derivatives, they highlight the potent HDAC inhibitory activity of certain analogs. For example, Quinazolin-4(3H)-one-based hydroxamic acids incorporating Heptanamide demonstrated sub-micromolar IC50 values for HDAC inhibition. [] Molecular docking simulations suggested that these compounds, particularly N-Hydroxy-7-(7-methyl-4-oxoquinazolin-3(4H)-yl)heptanamide (5b) and N-Hydroxy-7-(6-methyl-4-oxoquinazolin-3(4H)-yl)heptanamide (5c), exhibited strong binding affinity to the active site of HDAC2, surpassing that of SAHA (Vorinostat). [] This binding interaction is likely responsible for the observed HDAC inhibition.
Q2: What is the significance of the solid-solid phase transition observed in Heptanamide monolayers on graphite?
A2: Heptanamide exhibits a unique solid-solid phase transition at 330 K from a pgg symmetry to a p2 symmetry in its monolayer form on graphite. [] This transition is unusual among alkylamides of similar chain lengths, suggesting specific intermolecular interactions influenced by the Heptanamide structure. Further research could elucidate the exact nature of these interactions and their implications for potential applications.
Q3: How does the presence of an amide group influence the self-assembled monolayer (SAM) formation of N-(2-mercaptoethyl)heptanamide (MEHA) on gold surfaces?
A3: Compared to decanethiol (DT) SAMs, MEHA SAMs demonstrate enhanced thermal stability, attributed to the formation of internal hydrogen bond networks facilitated by the amide group. [] This finding highlights the role of specific functional groups in dictating the properties and stability of SAMs.
Q4: Can Heptanamide derivatives act as latency-reversing agents (LRAs) for HIV?
A4: Research suggests that some Heptanamide derivatives show potential as LRAs by inhibiting HDAC activity. Virtual screening identified four Heptanamide derivatives as potential LRAs. [] Subsequent in vitro studies confirmed that these compounds, including (S)-N-Hydroxy-4-(3-methyl-2-phenylbutanamido)benzamide (compound 15), N-(4-Aminophenyl)heptanamide (16), N-[4-(Heptanoylamino)phenyl]heptanamide (17), and 4-(1,3-Dioxo-1H-benzo[de]isoquinolin-2(3H)-yl)-N-(2-hydroxyethyl)butanamide (18), displayed either HDAC inhibitory activity or HIV latency reversal capabilities. []
Q5: How do different host plants influence the phytochemical profile of the parasitic plant Cuscuta reflexa?
A5: GC-MS analysis revealed distinct phytochemical variations in Cuscuta reflexa when grown on Cassia fistula compared to Ficus benghalensis. [] For instance, compounds like 1H-1,2,4triazol-5-amine-1-ethyl, D-glucitol-4-O-hexyl, 3,4,5-trimethoxy cinnamic acid, 3,6-dimethoxy phenanthrene, and 3,5-di tert butyl 4-hydroxyanisol were exclusively found in Cuscuta reflexa parasitizing Cassia fistula. [] Conversely, vanillin, 3-aminopyrrolidine, cetene, sarcosine N-isobutyryl-tetra decyl ester, 4-((1E)-3-hydroxy-1-propenyl)-2-methoxyphenol, 1,5-diphenyl-2H-1,2,4 triazoline-3-thione, 1-octadecene, heptanamide,N-(1cyclohexylethyl)-2-methyl, scoparone, and 3’methyl-2-benzyledine-coumaran-3-one were only detected in Cuscuta reflexa parasitizing Ficus benghalensis. [] These findings suggest the host plant plays a crucial role in shaping the parasite's chemical composition.
Q6: Has Heptanamide been used to create water-repellent coatings on textiles?
A6: Yes, a derivative of Heptanamide, specifically 2,2,3,3,4,4,5,5,6,6,7,7,7-tridecafluoro-N-[3-(triethoxysilyl)propyl]-heptanamide, has been successfully employed for creating protective hydrophobic and oleophobic coatings on various textiles, including cotton, wool, and polyester. [] This compound imparts impressive water repellency to the treated fabric, achieving a water contact angle of at least 120 degrees. []
Disclaimer and Information on In-Vitro Research Products
Please be aware that all articles and product information presented on BenchChem are intended solely for informational purposes. The products available for purchase on BenchChem are specifically designed for in-vitro studies, which are conducted outside of living organisms. In-vitro studies, derived from the Latin term "in glass," involve experiments performed in controlled laboratory settings using cells or tissues. It is important to note that these products are not categorized as medicines or drugs, and they have not received approval from the FDA for the prevention, treatment, or cure of any medical condition, ailment, or disease. We must emphasize that any form of bodily introduction of these products into humans or animals is strictly prohibited by law. It is essential to adhere to these guidelines to ensure compliance with legal and ethical standards in research and experimentation.